Glowworm Photomonitoring in the Waitomo Glowworm Caves, New Zealand
Waitomo Glowworm Caves, Tourism Holdings Limited1. School of Biological Sciences, University of Queensland2
Email: travis.cross@thlonline.com 1 ; d.merritt@uq.edu.au 2
Abstract
The Waitomo Glowworm Caves have the most visited glowworm display in the world. Prior to 2009, the glowworm monitoring program had been based on intermittent quadrat counts at two sites. Data from these quadrats was limited, not statistically robust, and difficult to interpret. One of the characteristics of the quality of the display is the extent of the colony’s coverage of the cave ceiling and walls so effective monitoring should encompass as large a proportion of the colony as possible.
Experimental long-exposure photomonitoring was developed in late 2008 and its design reported at the 2009 ACKMA conference. Data from the ensuing two years showed that monthly photomonitoring provided useful population counts, but a less labour intensive method with better resolution data was required to gain a proper understanding of glowworm population and biological cycles. Consequently, time-lapse glowworm photomonitoring was trailed in early 2011 and a permanent time-lapse photomonitoring system installed in July 2011. Photographs are taken at 30 minute intervals to match the frequency of temperature and humidity data collection by the automated climate monitoring systems. Despite some reliability issues, the glowworm time-lapse photomonitoring system has collected some interesting and useful data that has revealed previously unknown information about the glowworm population in the Glowworm Grotto.
Introduction
Tourists have been coming to see glowworms (Arachnocampa luminosa) at the Waitomo Glowworm Caves since 1889. In the following years, the caves became a popular tourist attraction, hosting 500,000 visitors per year during peak. For this reason, the glowworm population and the Waitomo Glowworm Caves are very important to the local Waitomo economy and region.
Glowworms are viewed at two main areas by tourists, the Demonstration Chamber where feeding lines are viewed and the Glowworm Grotto where glowworm lights are viewed from a boat (Figure 1). These displays are situated on the cave ceiling above the Waitomo Stream, which is a source of aquatic prey insects for the glowworms.
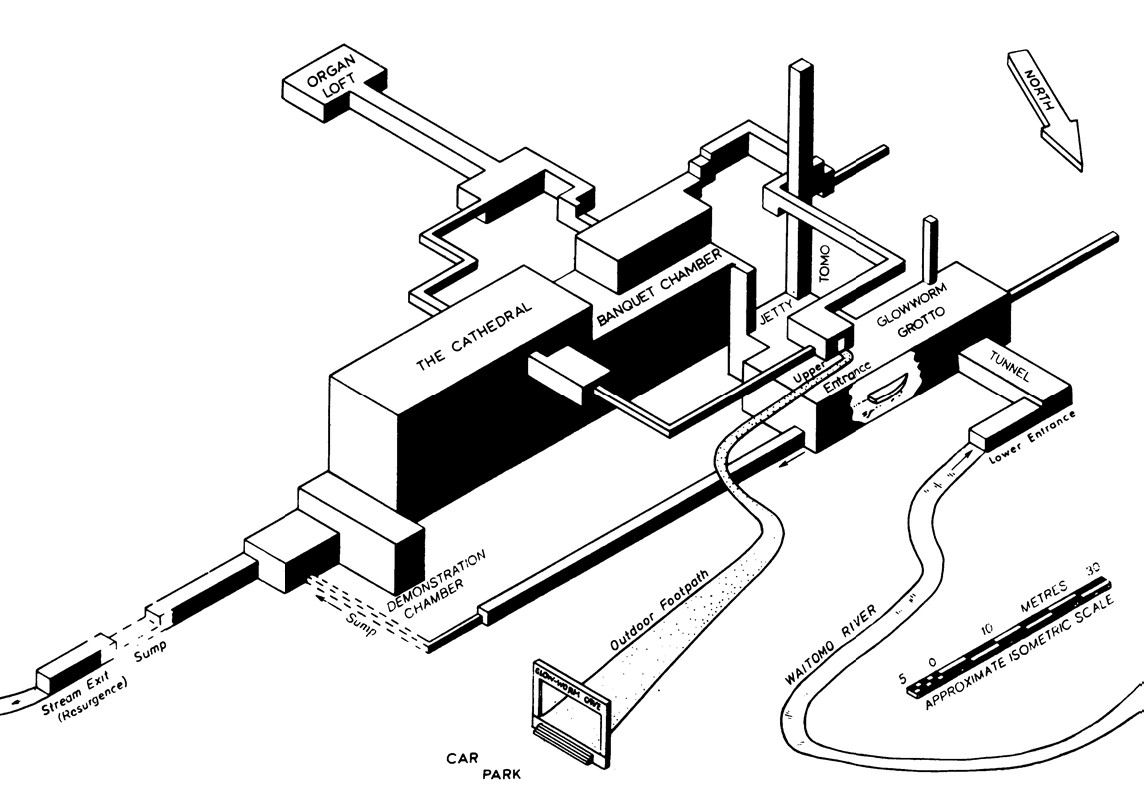
Figure 1. Isometric representation of the Waitomo Glowworm Cave with the Demonstration Chamber and Glowworm Grotto shown. (after de Freitas et al., 1982)
In 1979 glowworm numbers had declined so dramatically the cave had to be closed between April and July 1979. The decline was attributed to the replacement of the upper entrance door with an open grill door that allowed a free airflow through the cave in a process described by de Freitas et al., (1982). This caused glowworm desiccation and increased temperatures, which in turn increased occurrences of the glowworm-killing fungus Tolypocladium extinguens. Glowworms were transplanted from other caves to restock the population before the cave could reopen.
Between 1977 and 1980, C. Pugsley carried out photographic monitoring and quadrat counts at two-week intervals as part of his research on glowworm ecology (Pugsley, 1980, 1984). Quadrats counts were continued by Tourist Hotel Corporation staff (later Tourism Holdings Limited) but at intermittent intervals (D. Smith pers. comm. 2013). Until 2009, no regular, long-term monitoring of this important population was conducted, despite the installation of climate monitoring systems in the cave, primarily because of the difficulty of monitoring the glowworm population located on the high cave ceiling.
With the advent of affordable digital photography, glowworm photomonitoring was initiated during 2009 with several specific aims: 1) to determine annual changes in glowworm numbers; 2) to determine seasonal variation; 3) to provide early warning of any adverse effects of tourism or issues within the catchment. Monthly photomonitoring was done for a little over 2 years until it was decided a better method was needed and a method for continuous time-lapse photomonitoring was developed.
Monthly Photomonitoring
Photographic monitoring was done on a monthly basis starting from July 2009 by taking overlapping digital photographs of the main display in the Glowworm Grotto. A camera was attached to an extendable boom mounted on the cave wall (Figure 2). The boom was extended the same length each time, and then levelled to point straight up using a bullseye level placed on the lens. Then a series of 5 upward facing photographs taken through a plane parallel to the length of the Grotto at ~ 20º interval i.e. -40º, -20º, 0º (straight up), 20º & 40º with overlap between photographs. A Pentax E500 SLR camera with a 17.5-45mm lens was used with the focus set to infinity, ISO 800, F 3.5 and a 60 seconds exposure with the flash off. A wireless shutter release remote control was used to trigger each photograph.
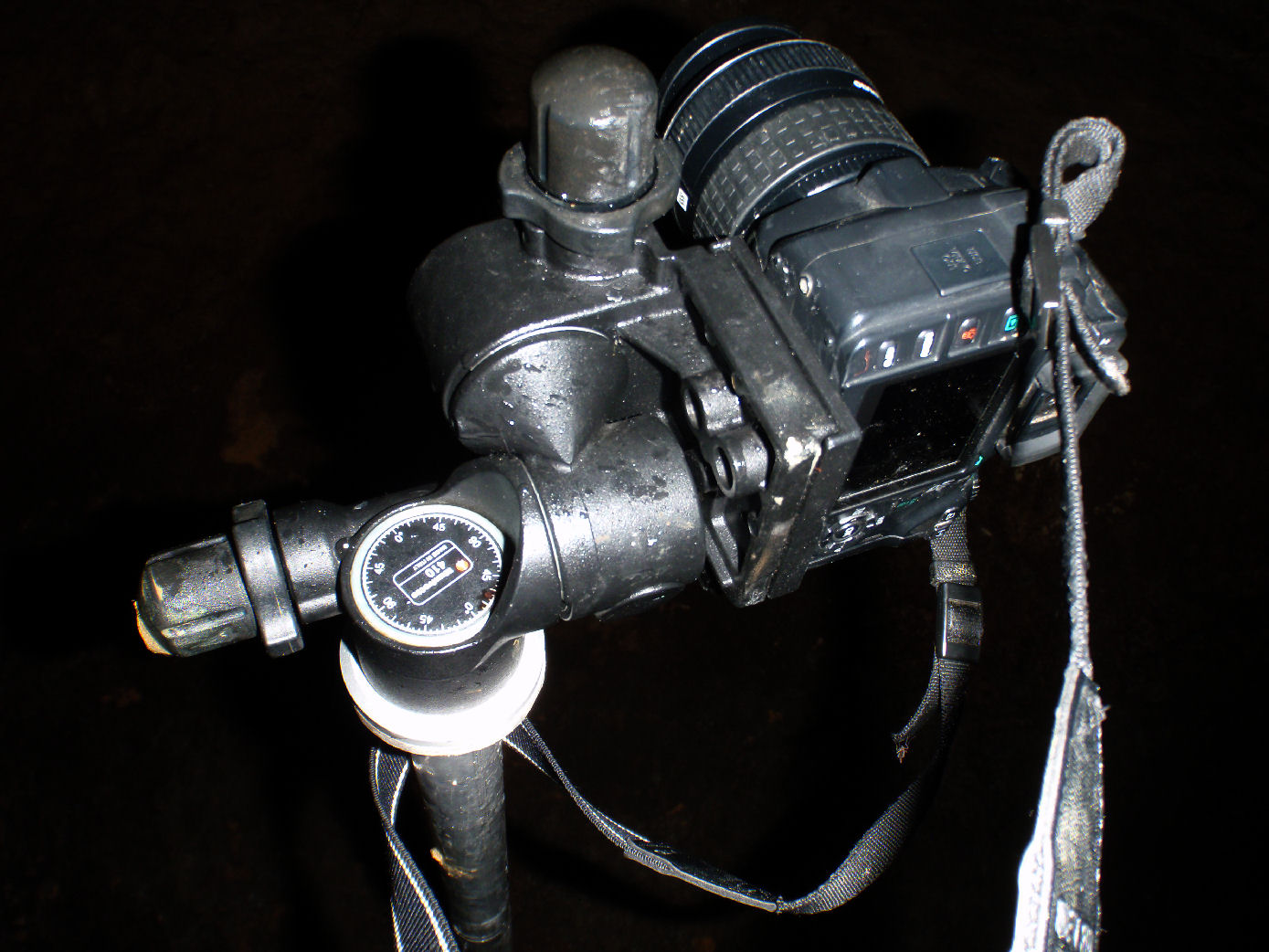
Figure 2. Monthly photomonitoring set up showing the camera attached to the boom and adjustable camera mount.
The resulting overlapping photographs were digitally stitched together resulting in a single photograph of the main Grotto glowworm population (Figure 3). This photograph was then analysed using the image analysis program, ImageJ to give the total number of glowing glowworms. Counts of up to ~ 3300 larvae were recorded using this method. Monthly data was collected for a little over 2 years and results graphed. Results were variable and showed a broad trend of declining glowworm numbers over time (Figure 4).
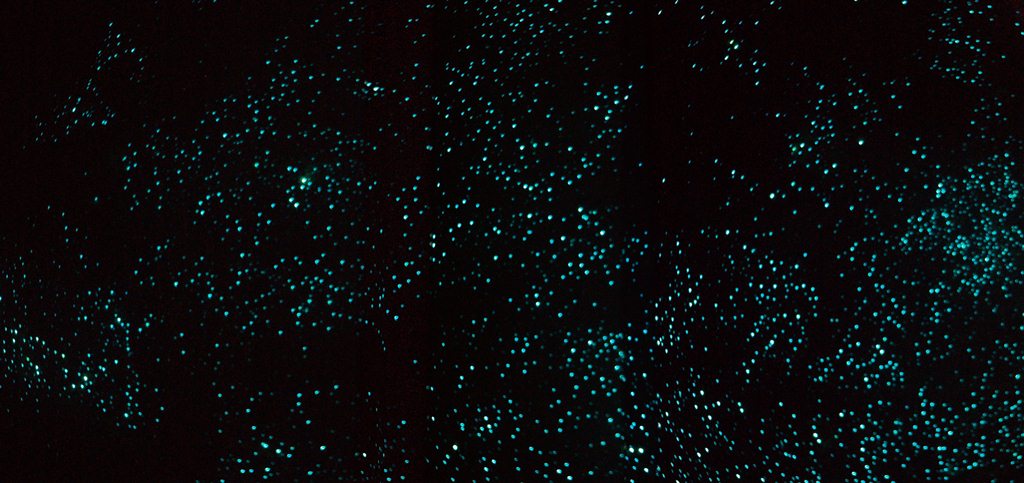
Figure 3. An example of a stitched monthly photograph showing the Glowworm Grotto glowworm display.
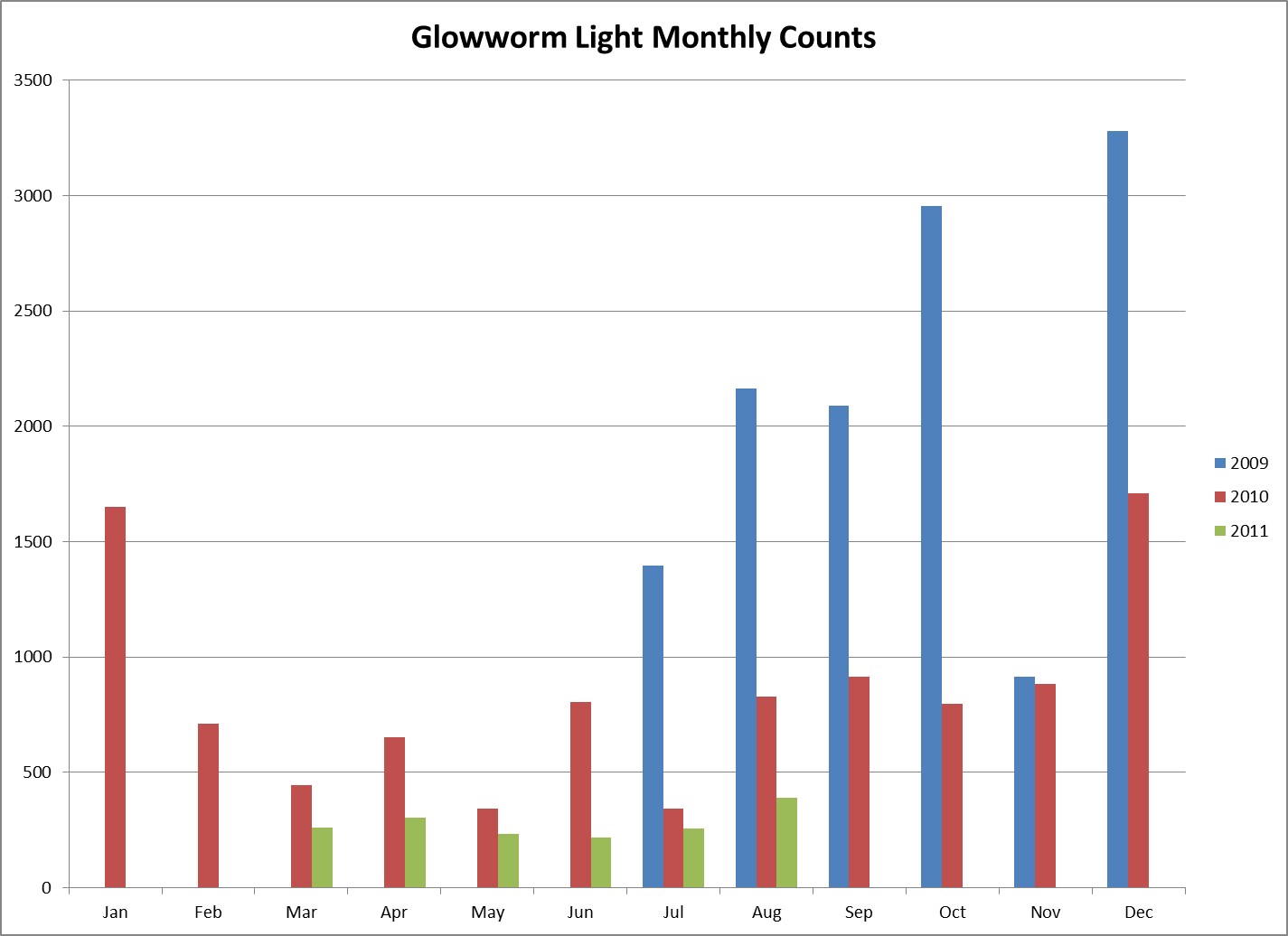
Figure 4. Monthly glowworm numbers plotted showing variable results and a broad trend of declining glowworm numbers over time.
In later times, two sets of monthly photographs were taken per session. The second set always yielded higher counts, possibly due to the glowworm population settling down after the light and sound disturbance of the equipment setting up. After a little over two years it was decided that results were of too low resolution to draw any real conclusions and a better method was required.
Time-lapse Photomonitoring
Time-lapse photography was first trialled in the Glowworm Grotto by Dave Merritt using equipment that had been tested in Mystery Creek Cave, Tasmania, for studies of Arachnocampa tasmaniensis bioluminescence rhythmicity. Following this successful trial, an off-the-shelf Habortronics time-lapse package was purchased and permanently installed above flood level on the true left, mid-way along the Grotto wall (Figure 5 & 6). Figure 6 shows the approximate time-lapse camera field of view. The system was based on a Canon Rebel T3 camera, powered by an 11.1 volt, 9 amp hour lithium ion polymer rechargeable battery that are exchanged monthly. At the same time the flash card with stored images was replaced.
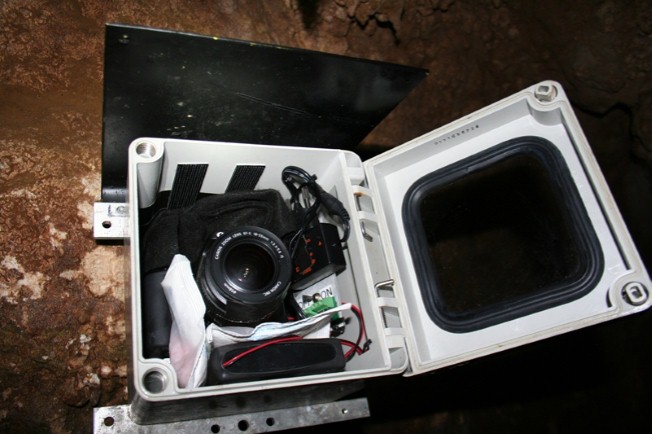
Figure 5. The Harbortronics time-lapse monitoring package installed in the Glowworm Grotto
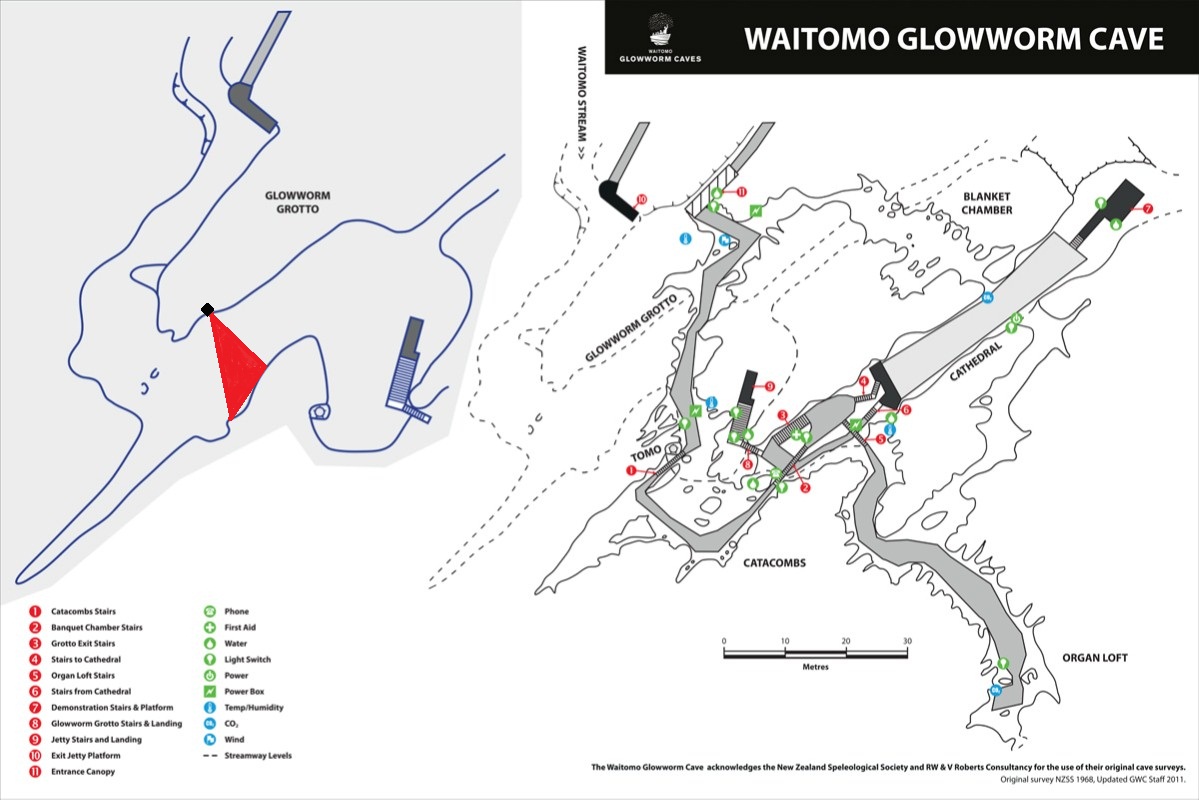
Figure 6. The Habortronics time-lapse camera location and approximate field of view shown in red on the enlarged section of the Waitomo Glowworm Cave map.
The time-lapse photomonitoring system took 20 second exposure photographs at 30 minute intervals to match the sampling rate of the automated climate monitoring system temperature and humidity readings (for automated climate monitoring system description see Cross, 2009). The sampling rate would allow correlations between glowworm light output and any potential environmental influences and would reveal any daily fluctuations in intensity. Although preliminary, the time-lapse glowworm photomonitoring system has yielded interesting information.
Results
The time-lapse photomonitoring system worked reliably for the first year of operation but developed ongoing battery and charging issues from late 2011, possibly due to the challenging conditions of high humidity and low temperatures. For this reason, the longest uninterrupted dataset is from January through to October 2011; thus, most of the data presented is derived from this time period.
A daily amplitude variation in overall intensity was present throughout the year. The amplitude of the daily cycle was much greater in summer than in winter (Figure 7). Removal of the daily cycle by calculating the mean daily light intensity showed a large reduction in mean light intensity from summer into winter (Figure 7). A correlation between mean daily Glowworm Grotto temperature and mean daily glowworm light output was also seen, with glowworm light output declining as temperature fell (Figure 8); however the annual change in temperature within the Grotto is relatively small (11 to 16 degrees C) compared to external daily and annual changes. In addition, a light intensity reduction appears to correlate with many, but not all catchment flood events (Figure 9).
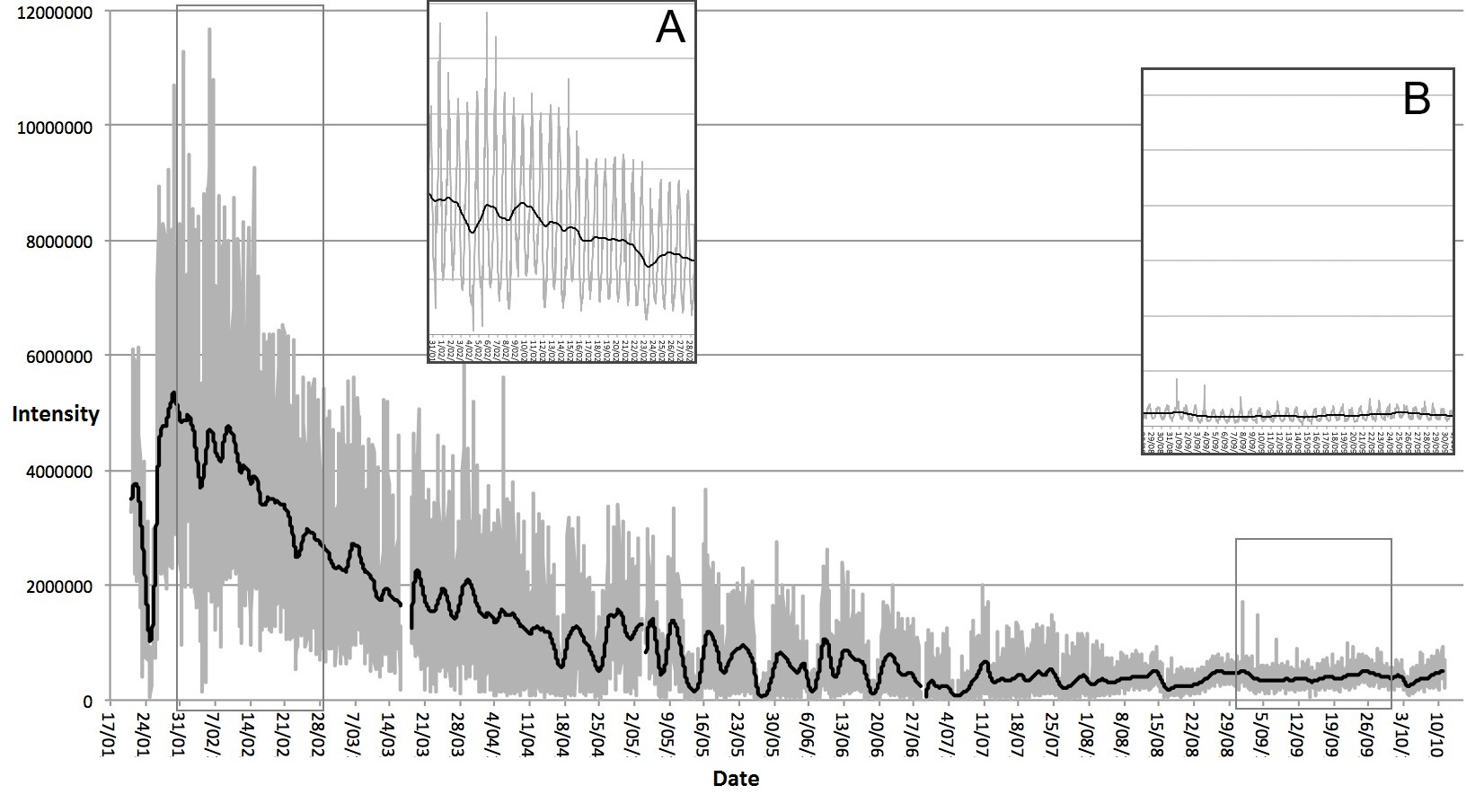
Figure 7 A large daily amplitude variation (grey) is seen between (A) “summer” (February) and (B) “winter” (September) and a reduction in mean intensity (black). The Y axis intensity unit is created by Image J and is the sum of the light of pixels in the photograph
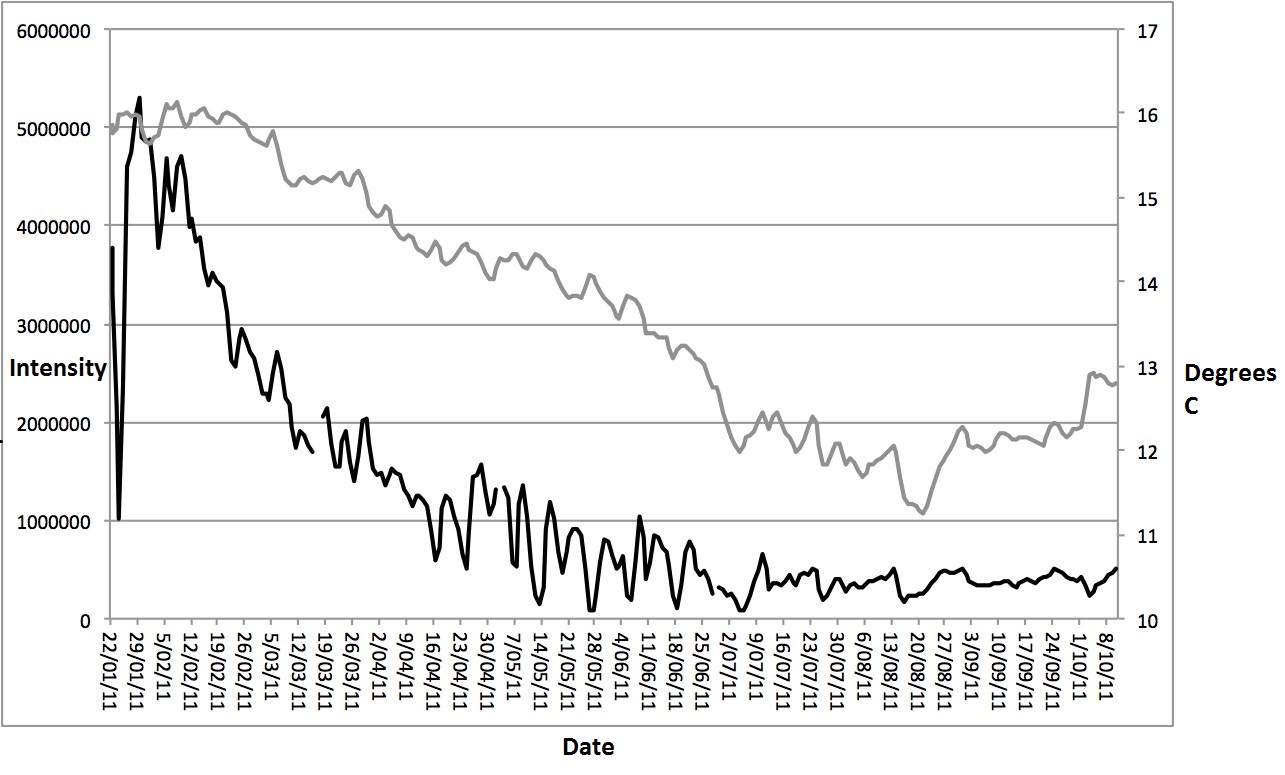
Figure 8. Continuous time-lapse data showing a correlation between mean glowworm light intensity (black) and mean Glowworm Grotto temperature (grey).
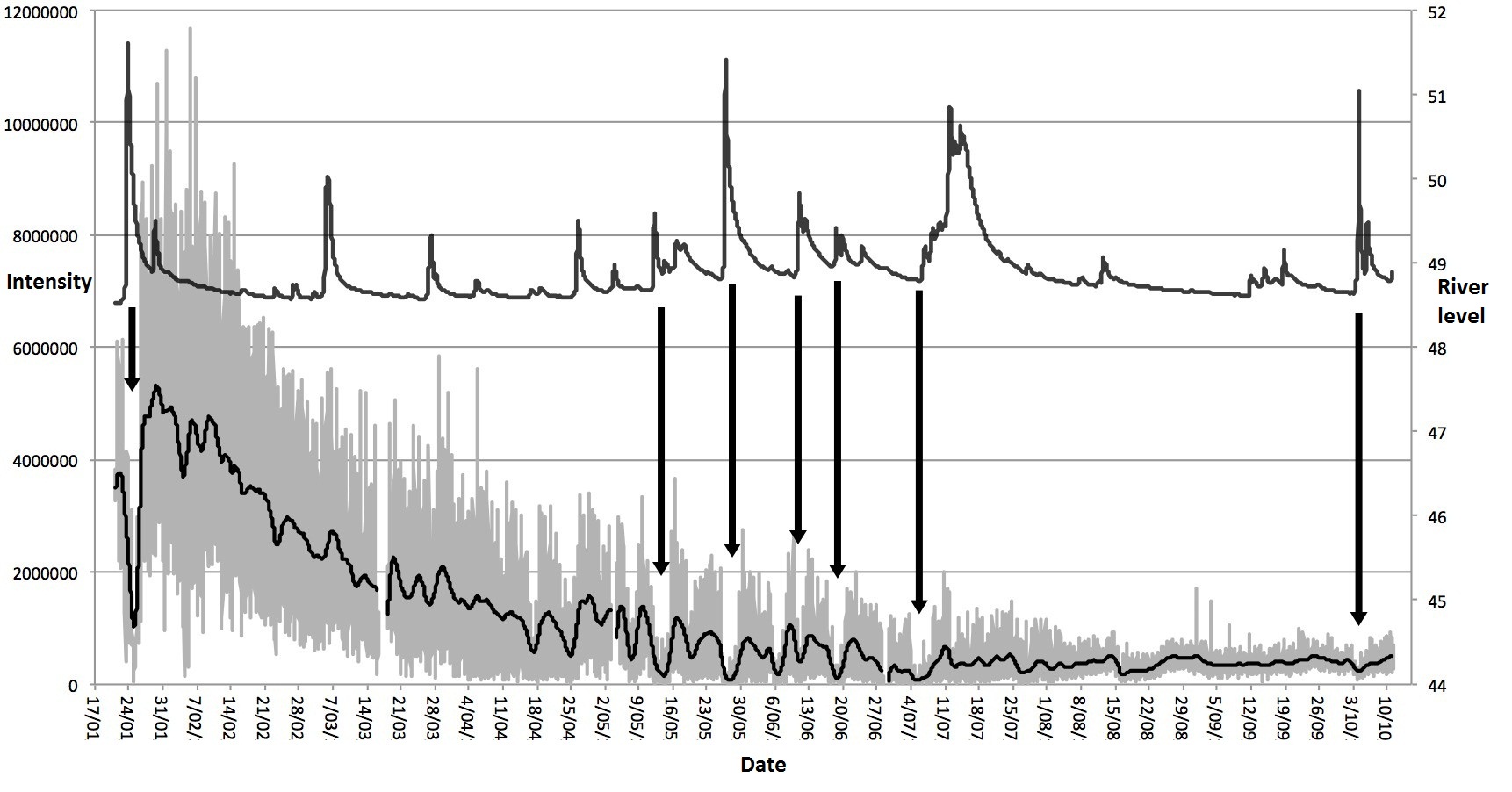
Figure 9. A comparison between time-lapse glowworm data and Waitomo Stream river level data (several kilometres upstream from cave) showing reduced glowworm light output in many but not all flood events.
Occasional unexplained sudden increases in light intensity and numbers glowing were seen in some cases, usually between 4 – 5 pm (Figure 10). This was thought to be due to disturbances in the cave. A consistent linear relationship was seen between number glowing and intensity apart from outlier disturbances mentioned above (Figure 11). The peak light output time of day appeared consistent between 4 and 6 pm from January to October 2011 whereas more variability was seen during 2012 (Figure 12).
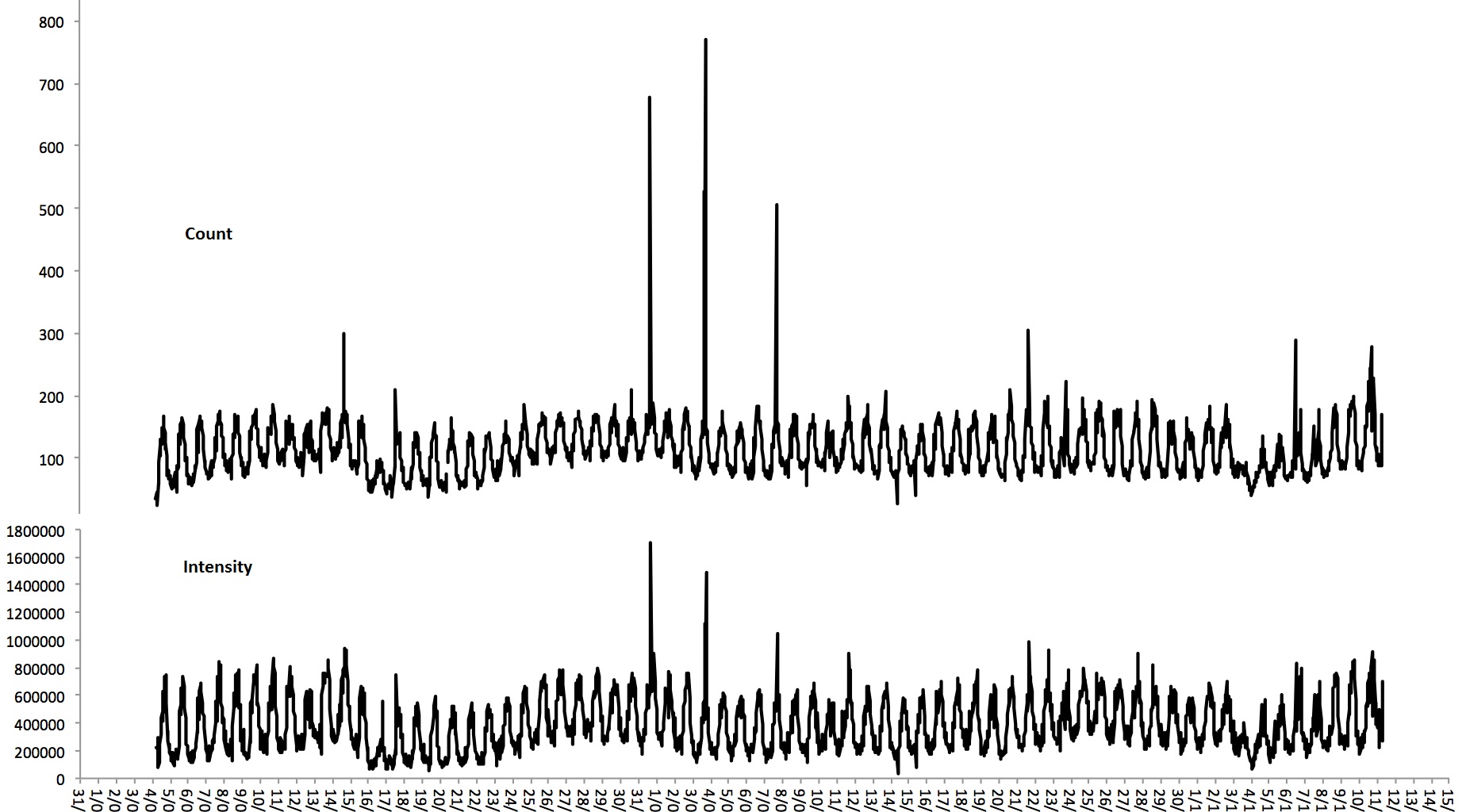
Figure 10. Sudden unexplained increases in light intensity and number glowing, usually between 4 and 5 pm.
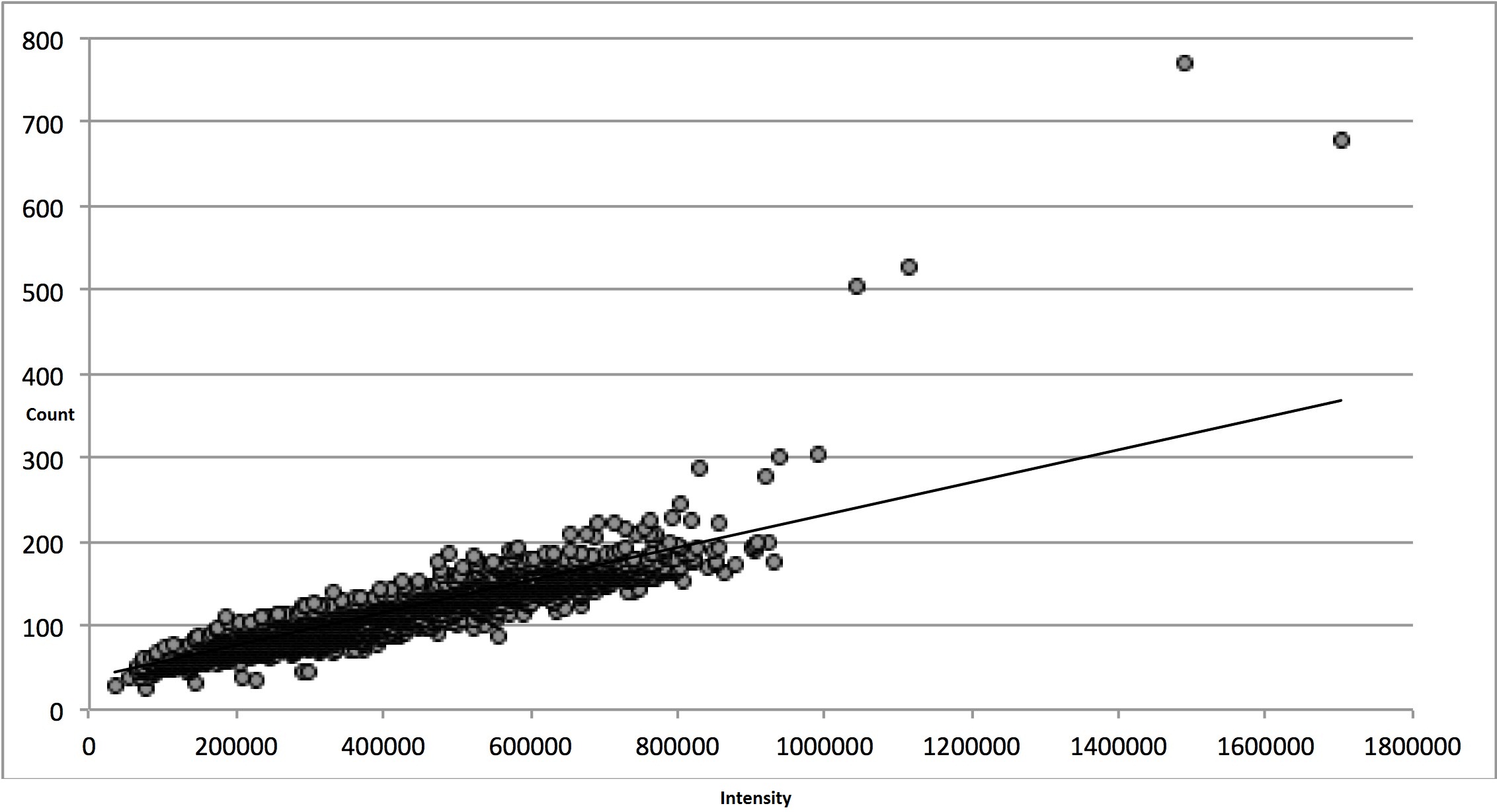
Figure 11. Time-lapse glowworm data shows a linear relationship between light intensity and number glowing.
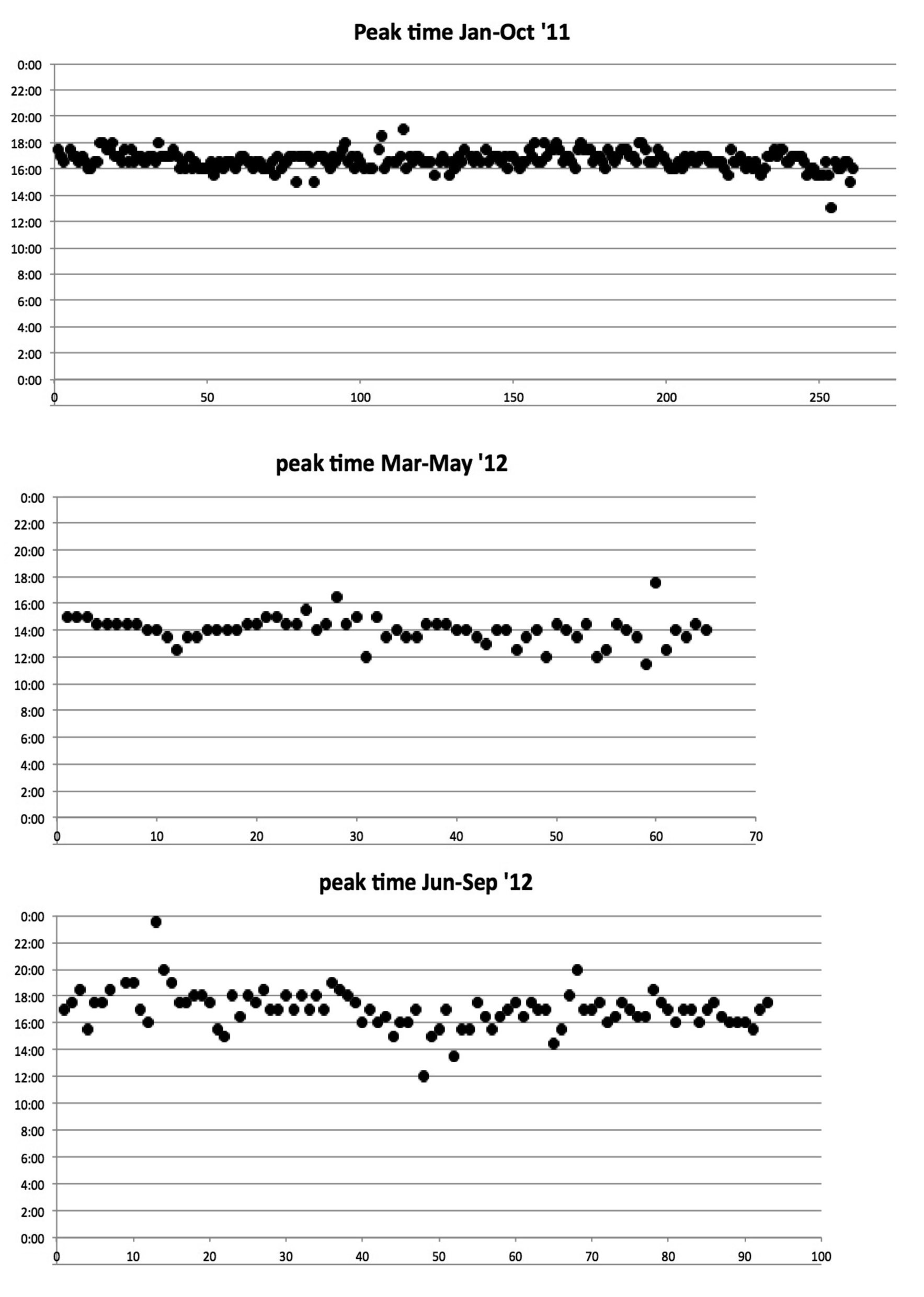
Figure 12. Time-lapse glowworm data shows relatively consistent peak light output time of day during 2011 but showed more variability during 2012.
Discussion
The pros and cons of monthly photomonitoring and continuous time-lapse photographic glowworm monitoring methods have become evident after using each for several years. Monthly monitoring captures the bulk of the Glowworm Grotto glowworm display but has coarse data resolution and is labour intensive. Time-lapse monitoring is automated and collects far higher frequency data that matches the capture of data from automated climate monitoring. Both methods require a similar labour component per month but the time-lapse camera provides much more fine-grained temporal data. This has allowed us to discover that the glowworms regulate their intensity on a 24 hour cycle, with the peak intensity in the late afternoon. On the down side, time-lapse monitoring currently only captures part of the Glowworm Grotto glowworm population and has proven to be subject to equipment malfunction in the tough cave environment. Also, data management and analysis has proven to be a challenge. Time-lapse imaging produces around 35 gigabytes of data per year, posing data storage and backup issues. The impact of the harsh cave environment on the time-lapse equipment became evident after many months in the cave. If the time-lapse system could be made more reliable, for example by heating the camera enclosure, or redundant systems could be installed, it would provide an informative monitoring system.
From the viewpoint of understanding the population dynamics of glowworms, light photomonitoring has a drawback in that it captures data on glowing larvae only, not the actual numbers present. Chris Pugsley showed in his study that under sub-optimal conditions, many larvae switch off completely and may switch on again when conditions improve. For a deeper understanding of annual cycles or long-term population density trends, the number of glowing larvae could be compared with counts of the number of larvae within quadrats. On the other hand, the photomonitoring provides information on the quality of the display, which is what visitors come to see, and allows us to measure the impacts of environmental variables such as flooding. It also allows us to assess the effect of changing any management practices.
Preliminary results suggest a correlation between temperature and glowworm light output. Whether temperature is directly influencing glowworm light output or indirectly by influencing prey insects or another unidentified factor is yet to be determined. River flood levels appear to cause a reduction in glowworm light output in many but not all cases so a better correlation is required before any conclusions can be drawn. The cause of sudden increases in glowworm light output and numbers glowing needs to be investigated. A cause could be a sudden noise created during guiding activities but is yet to be observed. The cause of a consistent peak time of day light output during 2011 but greater variably seen during 2012 has not yet been determined but could be influenced by many parameters that require further consideration.
Future Work
Given the dynamic changes in the intensity of the display that occur at daily, seasonal and annual scales, it is obvious that continuous and ongoing monitoring is required to understand environmental influences on the Waitomo Glowworm Cave population. Most pressingly, improvements in the reliability of the time-lapse camera are required. This could possibly be achieved by warming the enclosures and/or using an external power source rather than batteries. Photographic coverage of the whole Grotto is also desirable but would involve increasing the number of photomonitoring units. In the long term, it may be possible to predict the negative or positive impacts of environmental variables such as flood, rainfall and drought on the population by carrying out scientific studies that tease apart the direct affects of these variables on the glowworms from the indirect impacts on the glowworms’ prey. Our photomonitoring data will be a valuable resource for such studies. The most obvious requirement is to relate prey availability to glowworm population size. From the viewpoint of ensuring that cave tourism and cave management practices are not affecting the glowworm population, it would be informative to photomonitor the population in a wild, unvisited cave in the same catchment area.
References
Cross, T. (2009). Climate and Glowworm Monitoring in the Waitomo Glowworm Cave, New Zealand. Proceedings of the Eighteenth Australasian Conference on Cave and Karst Management.
De Freitas, C. R., Littlejohn, R. N., Clarkson, T. S. & Kristament, I. S. (1982). Cave Climate: Assessment of Airflow and Ventilation. Journal of Climatology: 2, pp 383-397.
Pugsley, C. W. (1980). Biology of the New Zealand Glowworm Arachnocampa luminosa (Skuse) (Diptera: Mycetophilidae) in Tourist Caves at Waitomo. Unpublished Phd thesis, University of Auckland.
Pugsley, C. W. (1984). Ecology of the New Zealand Glowworm, Arachnocampa luminosa (Diptera: Mycetophilidae), in the Glowworm Cave, Waitomo. Journal of the Royal Society of New Zealand: 14, (4), pp 387-407.