Karst Groundwater Systems: a brief overview
ABSTRACT
An introduction to karst hydrological terms and concepts is followed by a discussion of the factors that must be considered when classifying or studying karsts. These are then applied to a broad overview of the hydrology of the main Australian karsts.
Limestone Solution
There are two "magical" effects to be recognised when studying karst solution. I call them "magical" because the physical and chemical processes involved are complex, so for the non-scientist it is best to accept the effects at face value and concentrate on their significance to the way that caves and karst develops. Spate (1990) provides a simplified description of these processes and effects. Fuller details can be obtained from any standard karst text book (e.g. Jennings, 1985, Ford & Williams, 1989 or Gillieson, 1996).
The carbon dioxide (CO2) effect. The first magic effect derives from the importance of CO2 as the main factor effecting the ability of a given body of water to dissolve limestone (see discussion in Spate, 1990). The main sources of CO2 are biological processes which occur in vegetation and most importantly in the soil. The greater the CO2 content in the water the more limestone it can dissolve before it becomes saturated - we say that such water is aggressive. Thus rain water running over bare limestone and entering the caves immediately will have a low CO2 content (absorbed from the air) and be less aggressive than water that has soaked through the soil and picked up a high concentration of CO2. If water that is saturated with dissolved carbonate loses some CO2, as in seeping into a well-ventilated cave, it will become supersaturated and deposit the excess carbonate to form speleothems (secondary mineral deposits in caves). On the other hand, a water with low CO2 content, even though saturated with carbonate, may take up additional CO2 on entering a cave and so become aggressive and can dissolve pits in the ceiling, or corrode pre-existing stalactites.
Mixing Corrosion - the second magic effect - occurs whenever two different saturated karst waters meet and mix. By different we mean that they have different CO2 contents. For "magical" chemical reasons the mixture becomes under-saturated and aggressive again. Spate (1990) discusses the processes involved. This is a major factor in explaining why caves are bigger and more numerous at depth than near the surface - downward seeping water is mixing with the main body of groundwater at depth.
Karst Hydrological Zones
Figure 1 illustrates the terminology used to describe the zones within a karst water system, and the flow paths within the zones. At the top we have the epikarst which incorporates both the surface soil and the broken and fissured limestone immediately below it (the subcutaneous zone). Biological activity in the soil produces CO2 and strongly aggressive waters which dissolve the limestone surface below. These aggressive waters also enlarge the joints in the subcutaneous zone. However, as the downward flow is slow the waters quickly become saturated so that the zone of enlarged fissures is fairly thin. This epikarst zone is responsible for a large proportion of the solution that occurs in karst - but the result is surface lowering rather than cave development. The epikarst is a major storage area for rain water entering the karst system and can be regarded as an aquifer in its own right - perched above the relatively impermeable limestone below it. Some water leaks downwards through the tight cracks in the limestone but much of the epikarstic flow is horizontal and towards larger, isolated vertical conduits such as cave shafts (Figure 1).
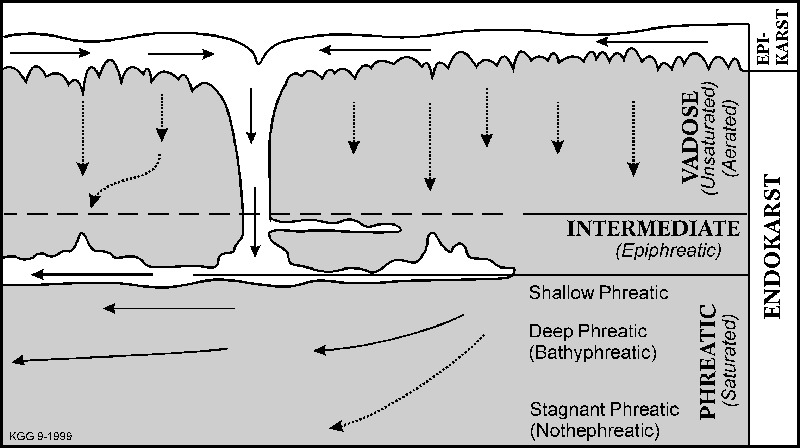
Figure 1 : Hydrological terms and flow directions in a typical karst aquifer.
The remainder of the karst water system is in the solid limestone rock and is referred to as the endokarst. This is divided into two main zones: the fully water-saturated zone known as the phreatic zone and the unsaturated, partly to wholly aerated, zone above that known as the vadose zone (Figure 1). In non-limestone aquifers the contact between these two zones is a well-defined water-table, but in karst areas this is more complicated and we find an intermediate and fluctuating zone of intermittent flooding sometimes referred to as the epiphreatic zone. This zone has characteristic of both the phreatic and vadose zones. The level of the water table or epiphreatic zone is controlled by the regional base-level, which may be sea level, or the level of springs at the down-flow edge of the limestone mass. By base level we refer to the lowest outflow point for the area. In fluvio-karst areas (ones where "normal" surface drainage occurs in conjunction with underground drainage) there may be local base-levels provided by the height of the surface valley floors.
Within the vadose zone water moves downwards, more-or-less vertically (depending on the orientation of the rock structure - most limestones have at least one set of near-vertical joints that water can follow). We distinguish between vadose seepage, where the saturated water moves slowly down from the epikarst through the relatively tight cracks, fissures, or pore spaces in the limestone; and vadose flow through solutionally enlarged cracks or still larger, open or soil-filled, conduits such as cave shafts and stream-ways. Where horizontal cave passages occur within the vadose zone, horizontal "stream" flow may occur - either as intermittent "flood stage" flows, or as more permanent "perched" streams which provide some of the distinctive and unpredictable character of karst aquifers. However, the zone of active horizontal cave development is lower - in the epiphreatic zone. These higher passages generally represent abandoned levels dating back to earlier higher levels of the phreatic and epiphreatic zones.
The intermediate, or epiphreatic zone is a zone of active solution, and the one in which most cave development occurs. It is also the zone of dominant horizontal water flow. The two go hand-in-hand. This is the zone where vertical vadose seepage or flow meets the main body of ground water and mixes with it. Thus we get cavities formed by mixing corrosion. Once a system of connected cavities is formed water can flow horizontally through these in the direction of the regional springs. This flowing water is constantly replenished and so, with time, can dissolve considerable volumes of limestone even though it might be relatively non-aggressive. For example, surface stream waters entering via a stream sink may have lower CO2 contents than the soil-derived waters of the vadose seepage, but much larger volumes. Strong flows can cause mechanical erosion as well as solution, and this is now seen as having considerable importance in the development of large river caves.
The phreatic zone is the body of permanently water-saturated limestone. It is a major reservoir of water, but except in the upper parts flow rates tend to be slow. The main water body tends to be saturated with carbonate and so is non-aggressive except where mixing occurs. This can be locally significant - as in coastal karsts where a body of fresh water may floats above, and mixes with, saline sea water; or where rising deep waters enter the limestone from below and mix with the phreatic waters. Rising hydrothermal waters that contain CO2 or sulphuric and other acids can also dissolve caves at depth. Within the phreatic zone we can recognise several sub-zones (Figure 1). The shallow phreatic zone is the uppermost zone characterised by relatively strong and horizontal flows just beneath the water table. In the deep phreatic or bathyphreatic zone water moves slowly in curved paths - downward at first, then rising again towards the spring outlets at the edge of the karst body. If the limestone extends deep enough there may be a relatively stagnant zone known as the nothephreatic zone.
Evolution of karst hydrological systems
The characteristics of a karst system evolve and change with time. The following is a generalised sequence for a typical karst region.
- Initial flows are slow and are diffuse (if the rock is porous) or in tight joints and bedding planes. The rock is mostly water-saturated with the water table close to the surface.
- With time, localised solution enlarges some of the joints or porosity. At a critical stage some flow routes become large enough to capture more water and flow faster and so develop at a faster rate than the others which stagnate. This localisation of flow into a few discrete paths is a characteristic feature of most karst aquifers.
- As conduits enlarge they can transfer greater volumes of water and the top of the saturated (phreatic) zone drops till it approaches that of the regional base level. The aerated (vadose) zone expands downward to replace it. The water table drops and may eventually become disrupted as flow is split into discrete flow channels. Downward flow from the surface is facilitated by the increasing permeability and any surface streams will be partly or wholly diverted underground. Thus the surface drainage is disrupted and eventually totally lost.
- Drained cave passages at higher levels may become unstable and be modified by collapse. Collapse may reach the surface to make major dolines.
- External factors such as sea-level changes, regional uplift and valley incision may complicate the sequence by creating multiple levels of solution. Local factors such as blockage by sediment fill may also divert water flow and passage formation into new areas.
Karst Aquifer Characteristics
Table 1: Characteristics of Karst Aquifers
Regional structure, boundaries and surface reliefClimate: wet, dry; tropical, temperate or periglacial.
Flow media: host rock porosity, bedding, jointing.
Flow type: diffuse(porous), fracture and conduit.
Recharge: diffuse or concentrated, local or exotic
Plumbing: Conduit network topology and storage
Outflow: spring response, base-level changes.
During any discussion of karsts from different regions it is important to remember that not only are karst aquifers different from conventional porous or fractured rock aquifers, but also that there are several quite distinctive types of karst aquifer. We must be wary of over-generalisation.
There are a number of factors that can vary, and which must be considered when comparing or classifying karst aquifers (Table 1). The following draws on recent reviews in Ford & Williams (1989), White (1988) and Gillieson (1996)
Regional structure, boundaries and topography
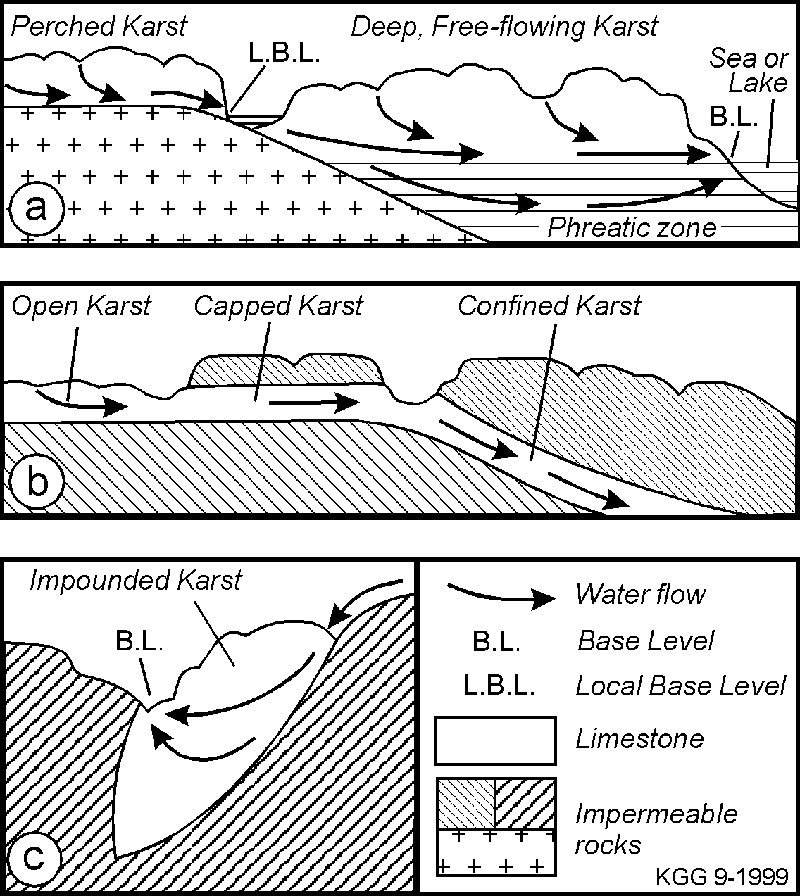
Figure 2: Some types of karst.
The overall size and shape of the limestone body and its relationship to adjacent, non-limestone, strata will effect the overall character of the karst drainage. The available topographic relief effects the potential depth of the water table - and hence the thickness of the vadose zone and provides the potential for the development of deep vertical cave systems in mountainous areas. White (1969) distinguished between perched karst aquifers where there is an impermeable barrier at shallow depth, and deep karsts where the limestone extends well below the regional base-level (Figure 2a). He also distinguished open karsts in which the limestone is open to the surface from capped ones in which there is an impermeable cover (Figure 2b); and free flow systems from those in which flow was confined and forced down below an impermeable bed (potentially artesian flows - Figure 2b) or where the flow was in thin limestone beds or lenses sandwiched between impermeable strata. Examples of the last type are common in the small east Australian karst areas, where Jennings (1985) referred to them as impounded karsts (Figure 2c).
Climate
The most obvious climatic factor would seem to be effective rainfall (Spate, 1999). For example the arid Nullarbor has a much sparser distribution of caves and surface karst (albeit with larger caves as possible relicts from an earlier climate) than the wetter Gambier Karst which has a similar limestone and structural setting (Jennings, 1967; Gillieson & Spate, 1992; Grimes & others, this volume). Temperature is also an important factor in distinguishing tropical karsts, with their distinctive surface landforms, from cooler karsts (e.g. Williams, 1978, Spate & Little, 1995) though seasonality of rainfall is also important. In southern Australia some karst areas show the influence of past periglacial processes (e.g. Jennings, 1967, Kiernan, 1990, 1995).
Flow Media
The character of the host rock will effect the flow. Limestone may be porous (more precisely, "permeable") or tight, it may be well-bedded or massive, it may be densely or sparsely jointed, and there may be larger-scale structures such as folds which will effect the movement of water within it. In karst aquifers we distinguish between primary porosity (pore spaces between the grains of the original sediment or primary fractures and joints in the original rock - Figure 3a) and secondary porosity which results from solution, or from subsequent fracturing and joint development in the lithified rock. Primary porosity tends to reduce with time due to compaction and cementation within the pores. This generally happens fairly early in the history of the rock and in many "hard-rock" limestone aquifers there is little primary porosity. On the other hand, in limestones secondary porosity increases with time as a result of the development of solution conduits.
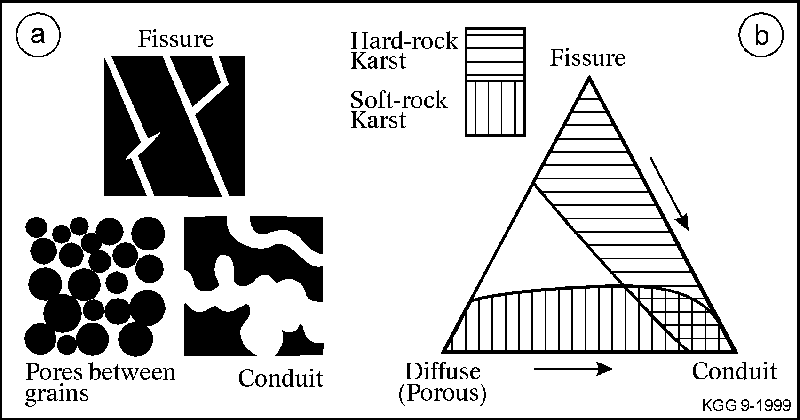
Figure 3: The three types of porosity (a) and associated flow possible in karst aquifers and their relationships (b). Arrows indicate the progressive change in flow character with time in typical hard-rock and soft-rock karst systems.
Flow Type
Traditionally, aquifer flow is divided into diffuse flow (porous rocks), fracture flow and conduit flow. Conduit flow has been considered typical of karst aquifers, but the three types are end-members in a continuum (Figure 3b). Many karst aquifers can have contributions from all three flow-types and the relative dominance can change with time as the aquifer evolves - as shown by the arrows in Figure 3b.
Good examples of changing flow character are provided in the "soft-rock" karst of the Gambier Karst Province (Grimes & others, this volume). During the development of the dune limestone karst of that region the character of the flow changes progressively with time. Initially it is entirely diffuse flow through the pores between the sand grains. As cementation develops this flow becomes more localised, and in time conduits will develop and may dominate the flow. Secondary joints and fractures may also develop in the cemented rock. Thus the flow character moves with time from the "porous" corner of Figure 3b towards the "conduit" corner.
Porous aquifers are characterised by laminar flow that can be isotropic (the water can move equally well in any direction) and typically show a well-developed water table and a slow response to input variations. Fracture flows are anisotropic (directional) and may be turbulent and faster if the fractures are open. Conduit flows are typically directional, turbulent, and "flashy". A recognisable water table may be missing in conduit aquifers.
Recharge
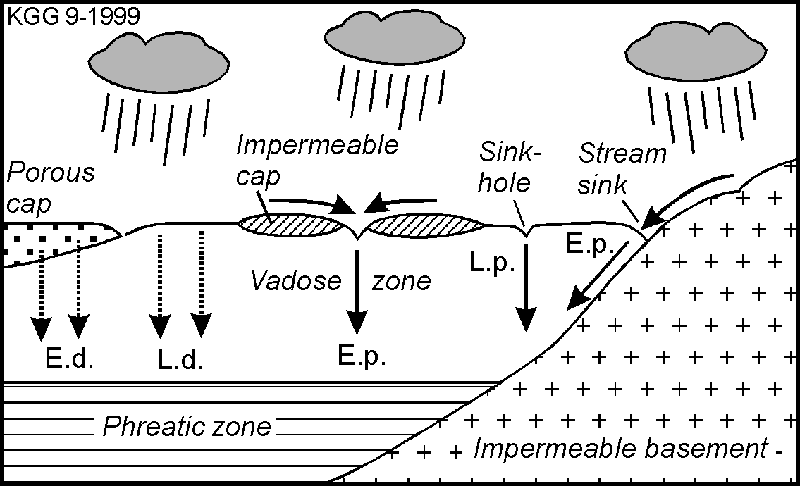
Figure 4: Types of recharge. (L.d. = Local (Autogenic) diffuse; L.p. = Local point input; E.d. = External (Allogenic) diffuse; E.p. = External Point input)
Recharge involves the amount of water input (i.e. the climate) and the type of input. We distinguish between local (autogenic) input from rain falling directly on the karst surface and external (allogenic) input from streams sourced in non-limestone terrain outside the karst area. We also distinguish between diffuse input (as where rain soaks into a porous soil cover) and point input via dolines and stream-sinks (Figure 4).
Small impounded karsts are dominated by external inputs. In more extensive karst regions autogenic input becomes more important. Point inputs favour the development of shafts and cave entrances more than does diffuse input. In syngenetic karsts (see later) the initial input is diffuse through the porous sand, but as a caprock develops flow becomes localised and vertical solution pipes then form. In calcareous dunes the lateral input of allogenic waters from adjacent swamps is an important factor in the cave development.
Plumbing & Storage
The karst plumbing involves the size, orientation and connectivity of the conduits and any associated porosity and fractures (Figure 5). It also involves the extent and character of storage within the system, including storage in the epikarst and in any rock porosity that exists. The nature of this plumbing is the most important control on the behaviour of the ground water, but unfortunately it is difficult to study directly as much of it is water-filled and too small for humans to enter. We have to rely on studies of inputs and outputs and flow-tracing between them, supplemented by extrapolations from the few cave passages we can enter and from point data from boreholes. Geophysics can provide some additional data.
The rock and flow type, and the location of inputs are major factors influencing the plumbing, but so also is the history of development of the karst - in particular any changes in the regional or local base levels. Changes in base level can produce complex stacked passage systems in which the higher levels provide important flood stage storage, and may also divert flood waters in quite unexpected directions (Kiernan 1990).
Outflow
Outflow occurs as point outputs such as surface or submarine springs, or diffusely into the sea or into adjacent non-karstic aquifers. The spring levels are controlled by the regional structure and contacts with adjacent impermeable rocks, or by sea level or valley-floor levels. This in turn defines the base level (Figure 2) and regional water table, which in turn affects the location of subterranean development of the cavities. The character of the outflow is determined by the karst plumbing and is an important clue to the nature of that plumbing. Thus springs in porous aquifers, or those with significant storage or long travel times will be steady - showing only limited responses to rainfall events at the input end. By contrast, conduit aquifers with limited storage or short flow paths will have springs with flashy responses.
Allowing for the differences and variability
Aquifers in porous karst rocks (such as those in the Gambier region) behave differently to those in the more "traditional" karsts in low-porosity limestones where flow is mainly in conduits. The latter tend to be much more complex.
Groundwater models, and approaches to studying and managing the aquifers, therefore must also differ. In porous karst we tend to map an actual water-table from bore-data and assume an even, diffuse flow over large distances with only minor local aberrations due to the secondary solutional pipes and fissures. We can map pollution by sampling over a regular grid and make reasonable guesses as to which way it will travel. However, it would still be unwise to assume that regional flow characteristics could be used to reliably predict the movement of a particular pollution slug - it might get into a local cave passage system and travel at a faster rate and in an unexpected direction.
A pair of local case histories illustrate this (Emmit & Telfer, 1994). Pollution from a cheese factory north of Mount Gambier was found to be moving to the south-west - along the regional flow direction. However, at another factory near Mount Schank the pollution moved to the northwest - at right angles to the regional flow, but along the regional joint-trend. In the latter case the flow must have been following conduits that had dissolved along the joints.
In the more traditional non-porous karsts a watertable may be an imaginary thing, whereas the concept of discrete "underground rivers" can be very real and perched water tables and streams that cross at different levels are definite possibilities (Figure 5). In these areas there is more emphasis on water-tracing, logging spring hydrographs, and other detailed site studies. At all times one must "expect the unexpected" and be highly suspicious of general theories or regional models when dealing with a specific local problem (e.g. Spate & others, 1976).
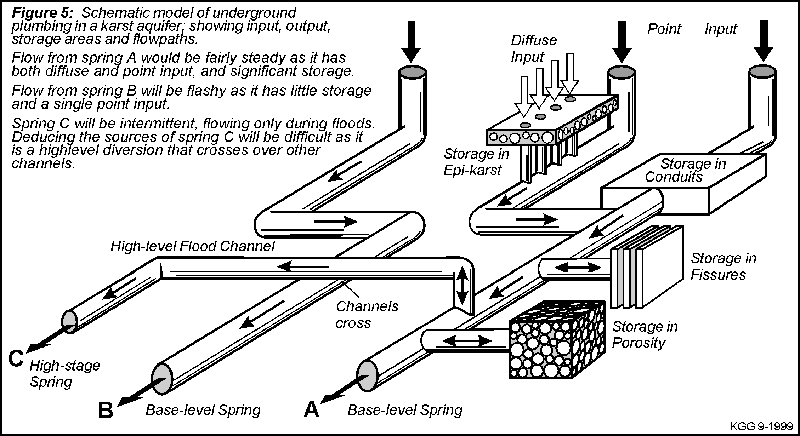
Figure 5
A summary of some of the techniques used in studying karst hydrology is presented in chapter 6 of Ford & Williams (1989) and chapter 2 of Gillieson (1996). More recent techniques have been discussed in a series of conference proceedings on engineering in karst (e.g. Beck & others, 1999).
The Karsts of Australia (a hydrological viewpoint)
In Australia we can see significant differences between, for example, the small impounded hard-rock karst
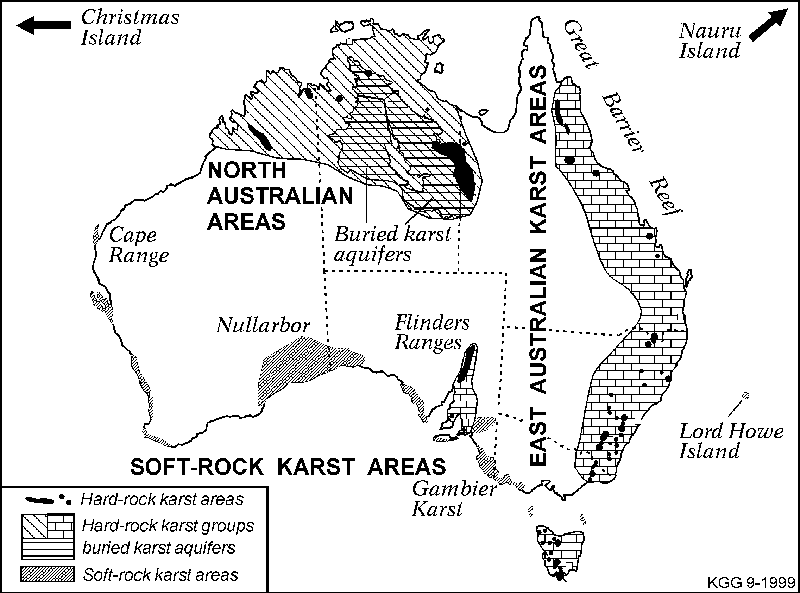
Figure 6: Major groupings of Australian karst areas.
Areas of the east-coast ranges, and the broader, porous soft-rock karst of the low-lying Mount Gambier area. A recent review of the Australian karsts is available in Gillieson & Spate (1998).
Bearing in mind the caveat about over-generalisation in karst - at a very general level Australian karst areas can be lumped into several major groups (see Figure 6):
Soft-rock karsts
The most distinctive group is the soft-rock karsts. This group comprises Tertiary marine limestones and Quaternary dune limestones of southern and western Australia. The climate is Mediterranean to sub-arid. They differ from the 'hard-rock' karst not only in being poorly consolidated, but also in having a pronounced primary porosity. The younger dune limestone show syngenetic features where the caves and karst features developed at the same time as the sediment was being indurated into a rock (Jennings, 1968; White, 1994). In both rock types recharge is local and diffuse, with minor point inputs through dolines. Allogenic input from surface streams is locally important.
The Tertiary limestones are extensive areas of soft, porous, horizontal-bedded limestone with some jointing. Examples include Cape Range (Allen, 1993), the Nullarbor (Lowry & Jennings, 1974; Gillieson & Spate, 1992), and the Gambier Karst (Grimes & others, this volume). With the exception of the Murray Basin, they are connected to the sea and their water-table history was influenced by Quaternary sea level changes coupled with local uplift and warping. Water flow is by both porous and conduit movement with some joint influence. Storage is large because of the large areas and the porosity and so the springs have steady flows.
The arid Nullarbor karst is the broadest of these areas (Gillieson & Spate, 1992). A low gradient watertable underlies the plain and its depth ranges from 30m in the north to 120m in the south. The ground water is brackish to saline - which complicates the cave genesis. The cave systems of the Nullarbor may have originated in a more humid climate phase in the late Miocene. Alternatively, they may have formed more recently by a combination of solution by saline waters, and mixing corrosion (James & others, 1993). This mixing was different to the usual case in which high CO2 vadose waters mix with low CO2 phreatic water - instead, in the Nullarbor we find fresh to brackish low seepage water mixing with the saline high CO2 groundwater, and the mixing occurs in several different settings (James & others, 1993). Since the onset of the present arid climate, the caves have been heavily modified by salt wedging and collapse processes.
The Quaternary dune limestones are soft syngenetic karsts (Jennings, 1968). They mostly form linear ridges parallel to the coast (see Bastian, 1964, 1991 and Williamson & Bell, 1980, for West Australia, and White, 1994 and Grimes & others, this volume, for southeastern Australia). Smaller areas occur on some of the islands of Bass Strait, and on Lord Howe Island (Standard, 1963 & Shannon pers.comm.). The calcareous dune sands are porous but as the sand becomes cemented solutional conduits become more important in the older dunes (c.f. Figure 3b). There is considerable storage available in the dune sands, but major through-caves can bypass this in places. In the Gambier Karst the dune limestones sit on the Tertiary limestone and the two are hydrologically continuous (see below, and Grimes & others, this volume). In West Australia some of the dune limestone is perched on an impermeable basement which channels flows into underground streams (Williamson, 1980, Williamson & Bell, 1980) whereas other areas overlie porous non-limestone aquifers with which they exchange water and the hydrology is more complex (Bastian, 1991). Lateral flow from swamps into the dune ridges can be a significant factor in cave genesis.
The Gambier Karst on which this conference was held is a good example of a soft-rock karst aquifer. It is described in more detail by Grimes & others (this volume). The Gambier Karst is hosted by a broad thick body of relatively soft and porous Tertiary limestone, overlain by Quaternary dune limestone ridges. At the regional scale it shows many characteristics of a porous aquifer; for example, it has a continuous, well-defined water-table and the springs show none of the "flashy" behaviour of conduit-driven karst aquifers (Holmes & Waterhouse, 1983). On the other hand the transmissivity in places is much higher than one would expect from the porosity of the limestone alone (Telfer, 1993, Emmit & Telfer, 1994), and at the local scale we see the typical irregular and unpredictable characteristics of conduit aquifers (see example given above from Emmit & Telfer, 1994). The aquifer is unconfined, deep, and open (uncapped). Outflow is via springs near the coast or offshore. Most water comes from rain and normally enters the aquifer diffusely through the porous surface sands, but some point input occurs via "runaway holes" in dolines (Herczeg & others, 1997). The redirection, by humans, of stormwater, farm effluent and other degraded waters into sinkholes has changed that picture to some extent. Some water enters from the allogenic Glenelg River, and further east in Victoria there are other allogenic rivers that rise outside the karst area but cross it on the way to the sea. Evolution of the karst conduits has been controlled by variations in the water table levels that resulted from the glacial fluctuations in sea level.
Hard-rock karst areas
The hard-rock karst areas can be split into two groups that are geographically and structurally distinct. Within those we can recognise several subdivisions.
The Eastern limestones comprise numerous small, impounded lenses of hard, non-porous, and well-jointed Palaeozoic limestones in a relatively strong topography (examples are given in Jennings, 1967, 1977, Dyson & others, 1982, Osborne & Branagan, 1988, and Webb & others, 1992, Kiernan, 1995, Bauer & Bauer, 1998). Evolution of these areas is influenced by uplift & stream incision (Osborne, 1993; Webb & others, 1992). Palaeokarsts of Mesozoic and Palaeozoic ages also occur (Osborne, 1984). The climate varies from tropical monsoon in the north to cool temperate in the south; and one could argue for a climatic subdivision of this group with a boundary line north of Brisbane delineating the distinctive tropical karsts (Jennings, 1982; Shannon, 1970, Spate & Little, 1995). The Flinders Ranges & Adelaide Hills of South Australia are broadly similar in structure to the east coast areas, but have a drier climate (Lawrence, 1997). An unusual magnesite karst occurs in northwest Tasmania (Houshold, this volume).
In detail, we find less consistency. In New South Wales the western areas are in a more subdued topography and the folding is less pronounced so the caves tend to be more horizontal and shallow (Osborne & Branagan, 1988). Water flow is dominantly through conduits with some joint influence, and water inputs are dominantly external from the adjoining non-limestone areas. Storage is limited by the small size, so springs tend to be flashy - although less so than non-karstic streams. The underground plumbing is variable and of limited extent in the small areas (eg Jennings, 1967, 1977), though a few larger areas such as Mole Creek in Tasmania with 150 km2 of exposed limestone have a more complex plumbing (Kiernan, 1990).
The North Australian karst areas are mostly medium to large areas of flat-lying limestone and dolomite. These are hard, with low primary porosity, and generally well jointed. The climate is tropical monsoon to semi-arid, and the surface karst landforms show strong similarities with the tropical northern part of the East Australian group (Spate & Little, 1995). We can distinguish two main subdivisions: the smaller but better-exposed areas such as the Limestone Ranges of the Kimberleys (Jennings, 1967, Williams, 1978) or the Gregory Limestone (Bannink & others, 1995); and the larger areas of mainly Cambrian carbonates in the Northern Territory and north-west Queensland (Randal, 1967, 1978; Grimes, 1988). These large Cambrian basins are poorly drained and largely buried under a cover of Mesozoic and Cainozoic sediments (shown as horizontal hatching on Figure 5). Much of the drainage in these basins is deep and probably in paleokarst that could date back to the Mesozoic or even earlier.
The plumbing and flow character is generally poorly known. Water flow is probably mainly in conduits with joint control, but some dolomites have diagenetic inter-crystalline porosity (Randal, 1978). The large basins have an extensive cover which varies from porous sands and laterite to impermeable black clay soils. Recharge is mainly from local rainfall with a diffuse input through the permeable cover or grikefields in the outcrop areas, but where the cover is impermeable clay then recharge is absent or restricted to scattered point inputs via large isolated dolines. There is some allogenic input from streams about the margins. In the smaller areas, such as the Kimberleys, there are major through-flowing external streams that have cut superimposed gorges across the karst (Jennings, 1967; Williams, 1978).
Coastal & Island Karst
This grouping overlaps with the soft-rock karsts described above, most of which are close to the sea. In addition, Australian territories include harder, Tertiary, reef limestones at Christmas Island (Grimes, in press) and Nauru Island (Jacobson & Hill, 1993), and there are submerged karst features on the Great Barrier Reef (Backshall & others, 1979). These coastal karst areas show a strong influence from sea-level controlled water table changes (complicated by uplift in some cases) and of mixing corrosion at the salt-fresh water interface. Salt-water intrusion after over-pumping can be a major problem.
Conclusion
Probably the most consistent aspect of karst is its inconsistency! Thus the prime rule of all karst studies must be to "expect the unexpected" and not to apply theoretical models until they have been verified by local detailed study. I have highlighted some of the factors which affect the behaviour of karst aquifers, and the way in which they vary between karst areas. Notwithstanding that variability, for convenience I have made broad groupings of Australian karst areas to simplify their description in general terms. However, the local plumbing of each area is distinctive, and must be treated as an individual problem.
And finally for the cave-oriented people among us, we must remember that the macropores, i.e. caves, are only a small part of the overall porosity of the karst aquifer. Most of the water moves through much smaller cavities, and a great deal of the underground fauna also inhabits these small inaccessible cavities (both flooded and dry) - they are beyond our reach, but not entirely beyond our ken.
ACKNOWLEDGEMENTS
Elery Hamilton-Smith started the whole thing with a suggestion that I give a 'short' introduction to the Water Below symposium! Andy Spate did a critical review of the draft text. Sue White also made useful comments.
REFERENCES
ALLEN, A.D., 1993: Outline of the geology and hydrology of Cape Range, Carnarvon Basin, Western Australia. Records of the Western Australian Museum,, Supplement 45, 25-38.
BACKSHALL,D.G., BARNETT,J., DAVIES,P.J., DUNCAN,D.C., HARVEY,N., HOPLEY,D., ISDALE,P.J., JENNINGS,J.N., & MOSS, R., 1979: Drowned dolines - the blue holes of the Pompey reefs, Great Barrier Reef. BMR Journal of Australian geology and Geophysics, 4, 99-109.
BANNINK, P., BANNINK, G., MAGRAITH, K., & SWAIN, B., 1995: Multi-level maze cave development in the Northern Territory. in BADDELEY, G., [ed] Vulcon Preceedings. (20th Conference of the Australian Speleological Federation). Victorian Speleological Association, Melbourne. 49-54.
BASTIAN, L.V., 1964: Morphology and development of caves in the southwest of Western Australia. Helictite, 2, 105-119.
BASTIAN, L.V., 1991: The hydrogeology and speleogenesis of Yanchep. in BROOKS, S., [ed] Proceedings of the 18th Biennial Speleological Conference. Australian Speleological Federation. 19-24.
BAUER, J., & BAUER, P., 1998: Under Bungonia. JB Books, Oak Flats. 284pp.
BECK, B.F., PETIT, A.J., & HERRING, J.G. 1999: [editors] Hydrogeology and Engineering Geology of Sinkholes and Karst, 1999. (Proceedings of the 7th multidisciplinary conference). Balkema, Rotterdam. 480pp.
DYSON, H.J., ELLIS, R., & JAMES, J.M., 1982: [editors] Wombeyan Caves. Sydney Speleological Society, Occasional Paper 8.
EMMIT, A.J., & TELFER, A.L., 1994: Influence of karst hydrology on water quality management in southeast South Australia. Environmental Geology, 23(2): 148-155.
FORD, D.C., & WILLIAMS, P.W., 1989: Karst Geomorphology and Hydrology. Unwyn Hyman, London. 601 pp.
GILLIESON, D.S., 1996: Caves: processes, development and management. Blackwell, Oxford. 324 pp.
GILLIESON, D.S., & SPATE, A.P., 1992: The Nullarbor Karst. in GILLIESON, D.S., [ed] Geology, Climate, Hydrology and Karst formation: Field Symposium in Australia: Guidebook, Special Publication No 4, Department of Geography and Oceanography, Australian Defence Force Academy, Canberra. 65-99.
GILLIESON, D.S., & SPATE, A.P., 1998: Karst and Caves in Australia and New Guinea, In Yuan Daoxian & Liu Zaihua [eds.], Global Karst Correlation, Science Press, Beijing PRC and VSP Press, Utrecht NL, pp229-256.
GRIMES, K.G., 1988: The Barkly Karst Region, North-west Queensland. 17th Biennial Conference of the Australian Speleological Federation. 16-24.
GRIMES, K.G., in press: The Caves of Christmas Island, Indian Ocean. 22nd Australian Speleological Federation Conference, Proceedings. (in press)
GRIMES, K.G., MOTT, K., & WHITE, S., this volume: The Gambier Karst Province. Proceedings of the 13th Conference on Cave and Karst Management, Mount Gambier. ACKMA, Melbourne
HERCZEG, A.L., LEANEY, F.W.J., STADTER, M.F., ALLAN, G.L. AND FIFIELD, L.K., 1997: Chemical and isotopic indicators of point?source recharge to a karst aquifer, South Australia. Journal of Hydrology, 192: 271-299
HOLMES, J.W. & WATERHOUSE, J.D.. 1983: Hydrology. In TYLER M J, TWIDALE C R, LING J K, and HOLMES J W, [eds] Natural History of the South East. Royal Society of South Australia, Adelaide, 49-59.
HOUSHOLD, I., this volume: Magnesite karst in Northwest Tasmania. Proceedings of the 13th Conference on Cave and Karst Management, Mount Gambier. ACKMA, Melbourne
JACOBSON, G.J., & HILL, P.J., 1993: Groundwater and the rehabilitation of Nauru. in McNALLY, G., KNIGHT, M., & SMITH, R., [eds], Collected case studies in engineering geology, hydrogeology and environmental geology. Butterfly Books, Sydney. 103-119.
JAMES, J.M. & SPATE, A.P., 1993: The role of sodium chloride in speleogenesis beneath the Nullarbor Plain, Australia. Proceedings of the 11th International Congress of Speleology. Beijing, China. 99-101.
JENNINGS, J.N., 1967: Some karst areas of Australia. in JENNINGS, J.N., & MABBUTT, J.A., [eds] Landform Studies from Australia and New Guinea. Australian National University Press, Canberra. 256-292.
JENNINGS, J.N., 1968: Syngenetic Karst in Australia. in WILLIAMS, P.W. & JENNINGS, J.N., Contributions to the study of karst. Australian National University, Department of Geography, Publication G/5, Canberra. 41-110
JENNINGS, J.N., 1977: Caves around Canberra. in SPATE, A.P., BRUSH, J., & COGGAN, M., [eds] Proceedings of the Eleventh Biennial Conference, Canberra. Australian Speleological Federation, Canberra. 79-95.
JENNINGS, J.N., 1982: Karst of northeastern Queensland reconsidered. Tower Karst, Chillagoe Caving Club, Occasional Paper, 4, 13-52.
JENNINGS, J.N., 1985: Karst Geomorphology. Blackwell, Oxford. 293 pp.
KIERNAN, K., 1990: Underground drainage at Mole Creek, Tasmania. Australian Geographical Studies. 28(2), 224-239.
KIERNAN, K., 1995: An Atlas of Tasmanian Karst. Tasmanian Forest Research Council, Hobart. 2 volumes.
LAWRENCE, R., 1997: Geology and caves of the Flinders Ranges. in WALSH, J., [ed], Proceedings of the 21st Biennial Conference of the Australian Speleological Federation. 93-111.
OSBORNE, R.A.L., 1984: Multiple Karstification in the Lachlan Fold Belt in New South Wales: Reconnaissance Evidence. Journal & Proceedings, Royal Society of New South Wales. 117, 15-34.
OSBORNE, R.A.L., 1993: A new history of development at Bungonia, N.S.W. Australian Geographer, 24(1), 62-74.
OSBORNE, R.A.L., & BRANAGAN, D.F., 1988: Karst landscapes of New South Wales, Australia. Earth-science Reviews, 25, 467-480.
RANDAL, M.A., 1967: Groundwater in the Barkly Tableland, N.T. Bureau of Mineral Resources, Australia. Bulletin 91. 111 pp.
RANDAL, M.A., 1978: Hydrogeology of the southeastern Georgina Basin and environs, Queensland and Northern Territory. Geological Survey of Queensland, Publication 366. 116pp.
SHANNON, C.H.C., 1970: Geology of the Mt Etna area. in SPRENT, J.K., [ed] Mount Etna Caves. University of Queensland Speleological Society, Brisbane. 11-21.
STANDARD, J.C., 1963: Geology of Lord Howe Island. J. Proc. Roy. Soc. NSW., 96, 107-121.
SPATE, A.P., 1990: Andysez No. 3: Carbonate solution made easy(ish). ACKMA Journal, 6, 24-25.
SPATE, A.P., 1999: Andysez No. 32: Morphoclimatic control. ACKMA Journal, 36, 40-42
SPATE, A.P., JENNINGS, J.N., SMITH, D.I., & JAMES, J.M., 1976: A triple dye tracing experiment at Yarrangobilly. Helictite, 14(2), 27-47.
SPATE, AP, & LITTLE, L., 1995: Is the conventional approach to karst area management appropriate to tropical Australia. in HENDERSON, K., HOUSHOLD, I., & MIDDLETON, G., [eds] Proceedings of the 11th Australasian Conference on Cave and Karst Management. ACKMA, Carlton South, 68-84.
TELFER, A., 1993: Groundwater resource management and community consultation - Blue Lake, South Australia. AGSO Journal of Australian Geology and Geophysics, 14(2), 201-206.
WEBB, J.A., FABEL, D., FINLAYSON, B.L., ELLAWAY, M., LI SHU, & SPIERTZ, H -P., 1992: Denudation chronology from cave and river terrace levels: the case of the Buchan Karst, southeastern Australia. Geological Magazine, 129(3), 307-317.
WHITE, S., 1994: Speleogenesis in aeolian calcarenite: A case study in western Victoria. Environmental Geology, 23: 248-255.
WHITE, W.B., 1969: Conceptual models for carbonate aquifers. Ground Water 7 (3), 15-21.
WHITE, W.B., 1988: Geomorphology and Hydrology of Karst Terrains. Oxford University Press, NY.
WILLIAMS, P.W., 1978: Interpretations of Australasian Karsts. in DAVIES, J.L., & WILLIAMS, M.A.J., [eds] Landform evolution in Australia. Australian National University Press, Canberra. 259-286.
WILLIAMSON, K., 1980: Stream caves of moist south-west Western Australia. in SAAR ,A., & WEBB, R., [eds] Proceedings of the twelfth Biennial conference, Perth. Australian Speleological Federation. 60-67.
WILLIAMSON, K., & BELL, P., 1980: The Augusta area, south-west of Western Australia: the reasons for its Karst Morphology. in SAAR ,A., & WEBB, R., [eds] Proceedings of the twelfth Biennial conference, Perth. Australian Speleological Federation. 53-59.