THE PHYSICAL MONITORING OF CAVES: THE DETERMINATION OF CAVE MODELS
ABSTRACT
Systematic measurement of physical variables is used to detect degradation of a cave's physical environment. An essential component of this system of protection is the development of a model of the cave. The cave model is used to determine the physical variables that are suitable for monitoring and also to determine the sensitivity of the system to proposed changes. The model development is a continuing process starting with intensive observation and measurement programs and maturing into a low maintenance activity. Examples of the components of this method are discussed.
INTRODUCTION
Environmental Monitoring can be seen as a process of protecting a vulnerable resource by measuring any changes in the resource, and then, if necessary, addressing the causes of the changes so that the resource can continue in its original state.
The monitoring of caves can be subdivided into categories as physical, chemical or biological monitoring. This paper is concerned with the physical branch of this subdivision, the monitoring of caves by making measurements of physical variables by physical methods. The boundary between physical and chemical is, as always, blurred. For instance, carbon dioxide in the atmosphere is connected to physical, biological and chemical phenomena, and it may be measured by chemical or physical methods, but whether it is measured chemically or physically does not matter, a physical, biological or chemical approach can be taken to examining the phenomena associated with it.
For the process of protection by the monitoring of caves to work, several components of the program must exist. First, it is necessary to make numerical measurements of appropriate parameters associated with the resource. When a change is found in one or more of these parameters then a model is needed to determine if the nature and size of the changes are significant.
The model, Figure 1, is a set of physical rules that have been determined as being an adequate representation of the physical system of the resource. The model has inputs, the stimuli that occur naturally or are imposed on the system, and outputs, which are the responses to those stimuli. Using the model, changes can be made to each input parameter until the changes in the model output have been matched to the changes in the resource and so the causes of the changes in the resource have been determined.
The model is also needed earlier in the process to determine which variables need to be measured. The model shows the sensitivity of the resource system to each of the variables, so that sensitive variables can be chosen for measurement.
Other essential elements of the process are sampling, model testing, and model evolution. Sampling is a statistical science of determining how many measurements are needed and where and when they should be made. Model testing involves comparing the behaviour of the model with the behaviour of the resource. Model evolution is the continual testing and improvement of the model by use of the measurements that have been made of the resource.
METHODS
In many environmental situations, the models are to be found in textbooks or are available as computer packages and there is little problem of model identification.
In caves the situation is different, there are many physical processes that may be operating in a cave and very little published work on model development. Each cave is likely to have a mixture of processes in it and be unlike any other particular cave.
The method of developing a cave model varies from case to case but my general approach is to inspect the cave for its shape and examine maps to locate obvious chimneys, hot traps, cold traps and other features that will control climate. The cave is examined for signs of drying, precipitation, freezing or flooding. A preliminary model of the cave is constructed. Locations are selected for making measurements and an instrument system is set up for a least one daily cycle.
The data is collected and examined to decide whether the cave responds to the daily cycle of variables in the same way as the preliminary model. The model is modified as required. The daily cycle monitoring is repeated at least 3 or 4 times in a year, and the model is modified as required to include the annual effects.
By this time the cave model should be fairly reliable, that is there should be a good ability to predict conditions in the cave.
DISCUSSION
A major step in starting to develop the model of a cave is the selection of the variables to be measured. Quite often the choice is limited by the instruments available with which to make the measurements.
Air flow is often an extremely important variable but the instruments that are available are often not suitable. The type of air movement that is most significant to cave climate is the net flow, over longer periods than minutes, often one hour is a good summing period. The air flow through caves often fluctuates in direction and intensity from minute to minute, but economical, sensitive, robust, integrating and direction sensitive anemometers are not available.
Air flow in a cave can be very slow and vague in large caverns and this makes measurement difficult so special techniques must be used to measure the air flow.
The measurement of the chimney coefficient and the reversal temperature require simultaneous measurement of conditions inside and outside the cave.
Outside wind and air temperature and humidity measurements require accurate sampling and measurement. Outside conditions may be more difficult to measure than the conditions inside the cave. This is at least partly because of the strong gradients of temperature, concentration and velocity that occur in the planetary boundary layer. The Stevenson Screen, the enclosure that is used at meteorological stations, measures air temperature and humidity about 2 metres from the ground, but the screen is sensitive to solar radiation and it does not accurately measure the condition of the air in the column that will affect the cave climate so local meteorological stations are of little use and other measurement systems must be established. Sampling problems can be a limit to accuracy when making measurements outside a cave. Temperature is often an easy measurement but it has technical problems in many situations.
Humidity can be a useful measurement, but most commercial instruments that can be connected to a recording system are inaccurate outside a cave and unable to make meaningful measurements inside a cave.
Other variables that can be measured are barometric pressure, air pressure differentials, stream flow, drip rate, water depth, dust fall, carbon dioxide concentration and radon concentration.
Model development may give problems in cases where some variables, such as high velocity air flow and the enthalpy of air over a wide temperature range, have a non-linear relationship to the other important variables such as pressure drop and temperature. This presents a problem for model development as even quite complex models that are linear are able to be treated by methods such as those described in reference [1]. But with non-linearity comes the possibility of chaotic behaviour, that is the response of the system is partly or completely independent of the stimulus. This is most likely to affect short term phenomena such as fluctuating air or water flow, but like many chaotic systems, parameters can often be found that are related causally, and although instantaneous air velocity may not be able to be predicted, the total air flow in an hour or a day may be quite predictable.
Non-linearity of relationships can also cause shock fronts in coupled heat and mass transfer phenomena and lack of symmetry in flow relationships.
COPPERMINE CAVE, YARRANGOBILLY
An example of a cave which would have a relatively simple model is Coppermine Cave at Yarrangobilly, Figure 2. Although a model has not been developed for this cave there is enough data available to make a tentative model. This cave is an outflow cave with a permanent stream flowing into the Yarrangobilly River. The higher level of the cave has a small airflow which is noticeable in a squeeze. Observations of this cave [ 2, 3, 4, 5] showed that the air flow through the cave followed a chimney model. When the internal air density is the same as the external air density there is no air flow. When the air outside the cave cools, the air density increases and air flows into the lower entrance and out of the upper entrance of the cave, as the more buoyant cave air rises. There is a very strong relationship between air temperature and cave air movement, Figure 3. The rate at which air flows depends on the shape of the cave and the temperature and humidity of the air inside and outside the cave.
The entrance section of the cave has a flowing streamway which will dominate the climate of that section of the cave, and the rest of the accessible cave has a climate dominated by the chimney flow.
Because of the chimney air flow, the cave will be sensitive to dust generation outside the cave entrances. The upper entrance has not been found but the lower entrance near the Yarrangobilly River will ingest dust and carry it into the cave. As the air velocity is low, large dust particles will settle and only fine dust particles will be carried deep into the cave. As the air velocity is low, the air motion will probably be laminar (not turbulent).
The air flow through the cave will cause what is termed "coupled heat and mass transfer" a combination of cooling or heating and wetting or drying. This is particularly powerful in winter when the air is cold, with a dew point temperature far below the cave wall temperature, and will cause very strong cooling and drying of the cave walls. As winter proceeds, the drying of the walls will extend further into the cave until, with warmer weather, the process reverses and the walls become moist again. The degree of drying of the walls depends very much upon the weather, a very cold spell will produce very strong effects.
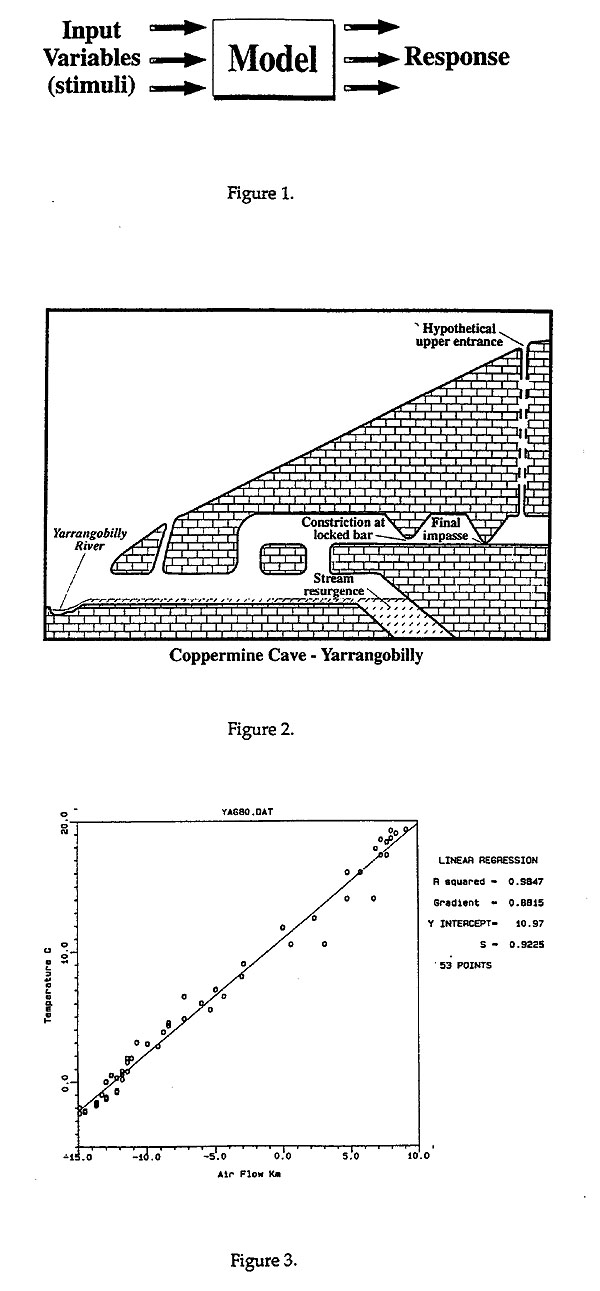
Figures 1,2 & 3
The drying process in winter is dependent on the weather outside the cave, but also on the chimney coefficient of the cave. This value can be measured by measuring the outside air conditions and the airflow through the cave. In Coppermine Cave it has a value about 0.3 m3K-1s-1, that is for each degree Celsius that the air outside the cave cools the air volume flowing through the cave will increase 0.3m3/sec. This is an important cave parameter, if any change was made to the cave, opening of new passages or gating of the entrance, this is the parameter that is in danger of changing, and which would cause the cave to dry out or change its climate.
Another important parameter is the reversal temperature, the temperature of the outside atmosphere for which air flow in the cave becomes zero. For Coppermine Cave this is about 11°C, but if the cave temperature changes with the seasons then this parameter will change too. If this parameter changes from its normal annual range then the distribution of temperature in the cave must have changed indicating that there has been a change in the physics of the cave. This may mean that other connections have been opened or closed. A change in reversal temperature might be the most sensitive indicator of changed climate in the cave.
THREATS TO COPPERMINE CAVE'S PHYSICAL ENVIRONMENT
There are many changes that could threaten the physical environment of Coppermine Cave. If the vegetation over the cave was changed or removed then the depth of roots and the level of biological activity in the soil over the cave could change, this could change the CO2 levels in soil, groundwater and dripwater in the cave and the CO2 levels in the cave, not to mention the concentration of calcium carbonate in the water. The upper entrance of Coppermine Cave has not been found and the air that flows through the cave may flow through the soil over the cave. A change of soil porosity could change the chimney coefficient of the cave, hence the temperature and humidity of the cave. Changing entrances and squeezes in the cave can also change the chimney coefficient and the reversal temperature, this includes the type of works associated with building gates. Change of vegetation near the entrance can change dust levels in the air and this air at times is ingested into the cave.
Changes in the hydrology of the catchment of the cave could change the stream flow or temperature and this would change the temperature of the cave. Changes to the road some kilometres distant caused heavy siltation in the cave some years ago, demonstrating the vulnerability to changes in the catchment area.
Changes in the cave's climate can affect the fauna of the cave, changing temperature, moistness and food supplies.
CONCLUSION
The development of a model is an important step in the protection of a cave when monitoring its environment. The choice of parameters for measurement is a compromise of what is required by the model and what is possible for economic, technical and practical reasons. The development of the model will be different for each cave and each model will continue to develop as more data is collected and more cave models become available for comparison.
REFERENCES:
[1] Kanasewich, E.R. (1981) Time Sequence Analysis in Geophysics. University of Alberta Press, Edmonton. Pp 480.
[2] Halbert, E.J. and Michie, N.A. (1979) Speleometeorology at Yarrangobilly Part V The Breeze in the Squeeze or the Climate of Coppermine Cave. Journalof the Sydney Speleological Society Vol. 23 No. 5 Pp. 125 - 134
[3] Michie, N.A. and Halbert, E.J.. (1980) Speleometeorology at Yarrangobilly Caves: Part VI Statistical Examination of Coppermine Meteorological Data. Journal of the Sydney Speleological Society Vol. 24 No. 5 Pp. 111 - 118
[4] Michie, N.A. (1982) Trip Report 9 - 12 April 1982 Yarrangobilly. Journal of the Sydney Speleological Society Vol. 26 No. 5 Pp. 88 - 89
[5] Michie, N.A. (1984) Determination of the causes of air flow in Coppermine Cave, Yarrangobilly. Helictite Vol. 22 No. 1 Pp. 21 - 30