Some months ago Dave Smith NZ (as opposed to Dave Smith OZ) sent me some information about websites dealing with earthquakes and caves. Including a new branch of science called 'palaeoseismicity' which apparently finds caves and their contents useful things. Did I ever say 'caves are the books in the library of the history of the world'? We will come back to palaeoseismicity later. Dave suggested that this would make a suitable subject for an ANDYSEZ (probably the last one) so I have been mulling over this for one for a while. Then we had the terrible tragedy of the Indian Ocean tsunami to remind us that earthquakes happen. This ghastly event rather put me off talking about earthquakes. Then our humble(?) editor sent me an article by Rolan Eberhard on Marakoopa Cave and recent earth movements. This article appears elsewhere in this issue - so I will add a little to Rolan's discussion.
Let's start with an anecdote - hopefully not too ill remembered - from Jewel Cave at Augusta in Western Australia. I think it was told to me by Ron Spackman - whom I have been unable to contact to check the facts. Perhaps someone in West Oz can confirm or deny the story or contact Ron to do so. And report back to us on the ACKMA list or a note in the next Journal.
I think it was the 1968 Meckering earthquake (Magnitude RM 6.9, MM IX - I will explain these below). It may have been the Cadoux in 1979 (RM6.2, MM IX)? These are 350 and 430km NNE of Jewel Cave. Anyway an earthquake shook the Jewel Cave kiosk - cracking the brick walls, refrigerator doors opened and items tumbled off shelves. Clearly this created some concerns amongst those in the kiosk - but some dozens of visitors in the cave had no idea an earthquake had occurred until they emerged to the shaken ice-cream purveyors.
Are caves good places to be in during earthquakes? Probably yes! Brick kiosks are amongst the worst places to be! Let's take another example. A RM 5.5 earthquake occurred right under Newcastle, New South Wales, on December 28 1989. This was the most serious natural disaster in Australia's history at that time claiming 13 lives, injuring more then 160 persons and leaving a damage bill estimated to be about A$4 billion, including an insured loss of about A$1 billion. The effects were felt over about 200,000km2 of New South Wales with isolated reports of movement from up to 800km away. Damage to buildings and facilities occurred within a 9000km2 region. In a number of web searches and trips to the Geoscience Australia library I have been unable to find any accounts of damage within the numerous coalmines in the region.
I am not claiming the research to be exhaustive but it is suggestive that there are no comments. Googling for 'earthquakes and mines' and 'earthquakes and caves' produces lots of hits but they relate to earthquakes produced by mining operations or to the aforementioned science of palaeoseismicity.
But Rolan and friends were in caves and felt, heard or observed (physical) effects of earthquakes whilst in caves. And a number of boffins are writing papers about using dating of broken speleothems to estimate the timing of earthquakes in the past. Which leads to the question why do we find broken speleothems in caves. Earthquakes might be one reason; cave visitors - human, scientists or other animals - are another. Erosion, settling of blocks by undercutting by water or by overloading of unstable piles by the steadily growing mounds of calcite might be other mechanisms. It would seem that the Broken Column in Jersey Cave at Yarrangobilly might well be attributable to earth movement as it predates human use, is on a stable floor and would appear to have been there for a very long time. Its shape suggests that vibrations might easily break it being slightly bulbous at the top and slender at the break point.
In contradistinction, the large broken stalactite that has speared into the mud of Calgardup Cave in Western Australia would probably only be detached by vibration or the weight of the calcite mass exceeding the strength of adhesion to the roof - or the strength of the overlying calcarenite rock.
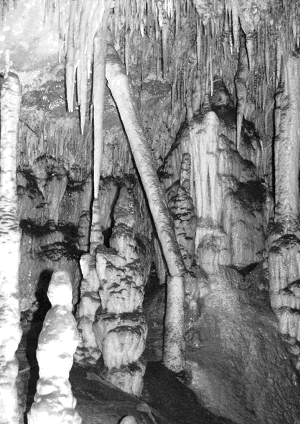
Broken Column, Jersey Cave, Yarrangobilly, NSW
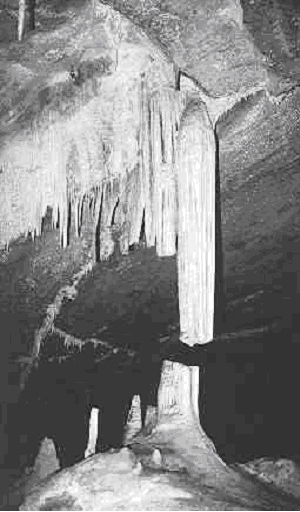
Broken Column, Lucas Cave, Jenolan
We can be more certain about the Broken Column, Lucas Cave, Jenolan. This will have been broken as the boulder pile on which it sits slowly settles as it is dissolved away by the water flowing beneath.
Perhaps we had better discuss earthquakes in more detail. As Ian Plimer puts it:
The rigid outer skin of this planet has been in perpetual motion for the past 4500 million years. This creates strain and the solid rocks bend. When the stress exceeds the strength of the rocks, they snap and move into new positions. During the breaking process, the energy release causes solid rock to vibrate and these vibrations travel through rocks as earthquakes.
The rigid outer skin of the earth in composed of plates which are pulling apart in mediation ridge areas and pushing together in other areas. This breaking of rocks causes earthquakes and the breakage in the rock is a fault.
The pulling apart of solid rock in mid-ocean ridge areas creates shallow earthquakes associated with mid-oceanic volcanicity. Associated with the pulling apart of the mid-ocean ridges are areas where one mass of rock slides past another, such as the San Andreas Fault in California.
The most intense earthquakes are at the margins of plates pushing together such as in Papua New Guinea, New Zealand, the Andes and the Himalayas. It is these earthquakes which release huge amounts of energy from shallow and deep breakage points and which have historically created massive devastation and large loss of life.
About 5% of earthquake activity is within the centre of stable rigid plates such as Australia. The area where the rocks actually break (the focus) is generally shallow in the earth's crust, normally less than 30 km from the surface.
[These latter 5%] are intraplate earthquakes. These are very difficult to predict because they do not lie in well-defined trends or patterns. And, because they are shallow, they are potentially very damaging to life and property despite the fact they are not as intense as earthquakes in well-defined earthquake zones. (p11)
The Cadoux, Meckering, Newcastle, and Tasmanian earthquakes mentioned above or by Rolan are these intraplate earthquakes. Later Plimer says:
When a rigid rock breaks, the energy is given out in a number of different vibrational wave forms. The fastest wave and the first felt is the P wave -- it is a compressional wave in rocks and behaves somewhat like a sound wave.
This is why many people would have noticed two shocks, the first being a P wave which arrives first and is associated with audible ground rumbles. [presumably these are the waves that Rolan felt]
Following the arrival of the P wave, is the S wave. This is a shear-type wave similar to a water wave. The difference in arrival times between the P and S waves can be used to calculate how distant the epicentre was.
Within a few kilometres of the epicentre of an earthquake, two other types of waves occur. These shock waves do not compress or shear the solid rock like P and S waves but actually make the solid ground move like a wave. These are devastating for any building, especially those supported by bricks, concrete or stone. (p11)
The two other types of waves are known as Love and Rayleigh waves. These are the ones that move rock masses - the ones that contain our caves. Love waves move the ground and rock mass from side to side horizontally and tend to knock buildings off their foundations because the building does not resonate (vibrate) in the same way as the ground does.
Rayleigh waves behave like a rolling ocean wave (as opposed to a tsunami wave). They tend to be extraordinarily destructive to buildings (and maybe caves?) because they produce more ground movement and take longer to pass.
Note the important point above - these are vibrations - the ground shaking. Where the surface materials are not bedrock - soil, alluvium, weathered rock - the things in them or on them shake themselves - sometimes to bits if the vibrations are strong enough.
This leads us to look into the ways the intensity (magnitudes) of earthquakes are reported. There are three scales used for reporting earthquake intensity so one has to be careful in interpreting what the press has to say about earthquakes - and the estimated magnitude are often modified in the weeks and months that follow an event as analysis proceeds. The three are the Richter (RM), Modified Mercalli (MM) and the newer Moment Magnitude scales. We won't consider the latter, which is based on the rock strength, the surface area of the rupture and the amount of rock displacement along the fault. It is not commonly used.
The Richter scale is an open-ended, logarithmic measure. Let's quote Trewby (2004 - not least to demonstrate to my colleagues across the Tasman that I do read about Enzed - even if it is this rather strange collection of facts):
The geologist, C.F. Richter, who lived in California, devised a scale to measure the strength of earthquakes. It is based on the magnitude recorded by seismographs, instruments used to measure vibration (the speed, or acceleration, of the ground as it moves suddenly). The Richter scale is logarithmic - each whole-number step represents a 10-fold increase in measured amplitude. Thus, an earthquake registering 7 on the scale is 10 times larger than one measuring 6, 100 times larger than a magnitude 5 and 1000 times as large as a magnitude 4. (p68)
2.0-2.9 Not felt by many; registered by sensitive seismographic machines 3.0-3.9 Slight vibration; hanging objects may swing 4.0-4.9 Vibration; small objects rattle and move 5.0-5.9 Furniture moves; masonry cracks and falls 6.0-6.9 Difficult to stand; walls and chimneys partially collapse 7.0-7.9 Buildings collapse; cracks in the ground; landslides 8.0-8.9 Damage to underground structures; rock masses move
To put the logarithmic business another way - and to incorporate some information from Plimer (1989):
Richter magnitude "Ground movement" ~ Number per year "Power" 1 1cm 70,000,000 0.5kg TNT 2 10cm 300,000 3 1m 49,000 4 10m 6,200 5 100m 800 6 1km 120 20 kilotonne atom bomb 7 10km 18 1 megatonne hydrogen bomb 8 100km 1 or 2 60 megatonne hydrogen bomb 9 1000km None recorded
Some comments on this table. "Ground movement" does not mean that an individual particle moves 1 cm or 1 km - I have given it to you as a means of trying to understand the size of the vibrations. "Power" - I know what a 0.5 kg block of TNT does as I have played with these blocks in an earlier career.
But I certainly do not know the differences between atomic or hydrogen bombs - but I guess we all know somewhat atavistically that they are big.
The atom bomb dropped on Nagasaki was 21 k tonne - that on Hiroshima 15-16 k tonne. The 'None recorded' was as at December 1989. Since then we have had two.
The Boxing Day 2004 earthquake had a magnitude of 9.0 (perhaps 9.3?). It was the largest earthquake since the 9.2 magnitude Good Friday earthquake off Alaska in 1964.
The Modified Mercalli scale is an intensity scale - somewhat subjective - with 12 steps designated using roman numerals (I to XII although the media sometimes translates these to Arabic numerals thereby creating potential confusion with the Richter scale. The scale looks like this:
Modified Mercalli Scale | ||
Average peak velocity (cm per second) | Intensity value and description | Average peak acceleration (g is gravity=9.80 metres per second squared) |
I. Not felt except by a very few under especially favourable circumstances. | ||
II. Felt only by a few persons at rest, especially on upper floors of buildings. Delicately suspended objects may swing. | ||
III. Felt quite noticeably indoors, especially on upper floors of buildings, but many people do not recognise it as an earthquake. Standing automobiles may rock slightly. Vibration like passing of truck. Duration estimated. | ||
1-2 | IV. During the day felt indoors by many, outdoors by few. At night some awakened. Dishes, windows, doors disturbed; walls make creaking sound. Sensation like heavy truck striking building. Standing automobiles rocked noticeably. | 0.015g-0.02g |
2-5 | V. Felt by nearly everyone, many awakened. Some dishes, windows, and so on broken; cracked plaster in a few places; unstable objects overturned. Disturbances of trees, poles, and other tall objects sometimes noticed. Pendulum clocks may stop. | 0.03g-0.04g |
5-8 | VI. Felt by all, many frightened and run outdoors. Some heavy furniture moved; a few instances of fallen plaster and damaged chimneys. Damage slight. | 0.06g-0.07g |
8-12 | VII. Everybody runs outdoors. Damage negligible in buildings of good design and construction; slight to moderate in well-built ordinary structures; considerable in poorly built or badly designed structures; some chimneys broken. Noticed by persons driving cars. | 0.10g-0.15g |
20-30 | VIII. Damage slight in specially designed structures; considerable in ordinary substantial buildings with partial collapse; great in poorly built structures. Panel walls thrown out of frame structures. Fall of chimneys, factory stack, columns, monuments, walls. Heavy furniture overturned. Sand and mud ejected in small amounts. Changes in well water. Persons driving cars disturbed. | 0.25g-0.30g |
45-55 | IX. Damage considerable in specially designed structures; well-designed frame structures thrown out of plumb; great in substantial buildings, with partial collapse. Buildings shifted off foundations. Ground cracked conspicuously. Underground pipes broken. | 0.50g-0.55g |
More than 60 | X. Some well-built wooden structures destroyed; most masonry and frame structures destroyed with foundations; ground badly cracked. Rails bent. Landslides considerable from river banks and steep slopes. Shifted sand and mud. Water splashed, slopped over banks. | More than 0.60g |
XI. Few, if any, (masonry) structures remain standing. Bridges destroyed. Broad fissures in ground. Underground pipelines completely out of service. Earth slumps and land slips in soft ground. Rails bent greatly. |
This comes from <http://www.eas.slu.edu/Earthquake_Center/mercalli.html>
Others describe XII as: Damage total. Waves seen on ground surface. Lines of sight and level distorted, Objects thrown upward into the air.
Note that the only mention in both scales of underground damage refers to underground pipes - nothing about caves and mines.
What probably happens is that caves and other cavities move with the rock mass - bits and pieces that might resonate (vibrate) like speleothems of partially detached roof or wall pieces at differing frequencies to the rock mass may well break off depending on their fragility and/or the magnitude of the Love and Rayleigh waves. As usual there will be a continuum of responses. For one there would be no doubt that caves in the hard Palaeozoic limestones of Tasmania will behave differently to the young calcareous aeolianites of Western Australia.
Those wishing to better understand earthquakes and estimates of their power might like to consult <http://
Turning again to this palaeoseismicity stuff. Forti (2004) suggests that there are several types of stalagmite breakage along sub-horizontal planes. He states that this is an unusual type of breakage and that it is produced by resonance induced by high-frequency seismic waves. The breakage is consistent in certain directions. If enough dates are available for broken speleothems and if there are groups of dates these will almost certainly reflect seismic events. He also point out that if there are tilted stalagmites - especially if the have erect tops - these may well reflect earthquake happenings - and provide excellent opportunities for obtaining good dates for the events.
Forti (2004) cites work by himself and Postpischl where they looked at two gypsum caves and reconstructed the seismic history of the Bologna region in Italy over the last 1200 years with an average error of +/- 11 years. This must have required a very large (and expensive) number of samples to analyse. He points out there is only about 2000 (perhaps 3000) years worth of historical record available to us but seismic history might be obtainable going back 500,000 years or more using U/Th and other longer term dating methodologies.
Other authors are not so sanguine. As Forti (2004) says:
The stalagmite itself is considered to be a homogeneous cylinder, perfectly connect to the floor, with its breakage being caused by resonance induced by the earthquake, and the diameter/height of the broken stalagmite being related to the horizontal acceleration of the seismic waves (which, in turn, is affected by the distance from the epicentre and the magnitude). Although this appears to be simple from a theoretical point of view, performing such a study is very complex because real stalagmites are very difficult from the model of the homogeneous cylinder, perfectly attached to the floor of the cave. (p 565)
This would seem to be a very considerable understatement!
Lacave [nice name!] and various et als in recent years (2000, 2002, 2004) have shown a bit more caution in regard to value of the conclusions which might be reached from speleothem-related palaeoseismic investigations - as have other authors and web sites that I have researched on your behalf. Very few of these sources have said anything much about earthquake magnitude estimates derive from cave-based investigations relying on such a 'reasonably strong' or 'moderately strong' quakes.
Here are their lightly edited conclusions and abstracts for you to consider:
Lacave et al (2000) - Conclusions
Most speleothems do not suffer dynamic amplification phenomena, because their natural frequencies are higher than the seismic frequency range (around 0.1 to 30 Hz). Only very elongated and thin speleothems ... could undergo such amplification that might lead to their rupture.
A natural frequency which is higher than the seismic excitation range means that the speleothem moves as a rigid object together with its basement. It is in this case subjected to inertial forces, which correspond to the ground acceleration multiplied by the speleothem mass. Consequently, the speleothems must break at their roots and then could give an indication of the peak ground acceleration. Most of the broken speleothems that can be observed in caves show a rupture in different part which could be on a heterogeneity of the structure itself ... Only very elongated speleothems could give information on the frequency content of past earthquakes.
Peak ground acceleration alone is a bad indicator of the magnitude of an earthquake, it would therefore be necessary to find traces of broken speleothems in a whole area in order to evaluate the damage extent. On the contrary, the inverse approach, which consists in excluding strong earthquakes in a given region, during the "life time" of present speleothems, seems to be more promising.
Lacave et al 2002 - Discussion and conclusions
It is interesting to compare the results obtained with the history of regional seismicity that is known - as far as strong events are concerned - for about 700 years. In fact, the only strong event known for that period is the 1356 Basel earthquake. Its epicentre was about 40 km away from the Milandre cave, and its magnitude (Ms) was probably between 6.5 and 7 [Richter]. According to Ambraseys et al, a mean value for the larger component of the horizontal PGA [Peak Ground Acceleration] of 0.7 m/s2 (Ms = 6.75) would be expected in this case on rock outcrop. Since the Milandre cave is situated about 40m below ground level only, this value would also approximately be valid in the cave. ... actually occurred PGA value may have been also significantly higher. This is even probable. It is known ... that the isoseismal counters were elongated in the direction from the epicentre to the region of Milandre, i.e. the attenuation in this direction was significantly less than the mean. Furthermore, both publications show Milandre within the IMSK = VIII isoseismal counter. This makes a PGA-value of 1 m/s2 and even 1.5 m/s2 much more probable than a value of 0.7 m/s2.
The main tentative conclusions that can be drawn so far are:
- Most of the existing stalactites (and stalagmites), except long and slender ones, hardly break during realistic earthquakes, say with PGA < 1 g.
- There are many broken stalactites present in the pilot cave where low or very low seismic vulnerability is inferred for their estimated unbroken shape. It seems therefore improbable that the majority of them broke during a seismic event.
- Most of the long and slender stalactites are expected to break during a "reasonably" strong earthquake, say with 0.3 g < PGA < 1 g.
- The observed data nevertheless indicate that probably at least one seismic event has occurred. (As long as no datation [sic =dating] of the broken stalactites is undertaken, multiple events cannot be distinguished from a single event.) The most probably attained PGA was of the order of 1 to 2 m/s2 ... The co-existence of intact, but vulnerable stalactites with broken ones of very low vulnerability is not necessarily a contradiction. As long as no datation has been undertaken, it is possible that the unbroken vulnerable stalactites are younger than the last strong earthquake that had broken the less vulnerable ones.
Lacave et al (2004) - Abstract
Is it really plausible that earthquakes break speleothems? May unbroken speleothems prove that no strong earthquake has ever occurred during a certain period of time? The mechanical behaviour of speleothems has been investigated through static bending tests performed on stalactites and soda straws. These tests give an indication not only of the mean tensile resistance, but also - more importantly - of its variation. In fact, it is this variation that makes it difficult to estimate the acceleration necessary to break an individual speleothem. That is why a statistical approach is mandatory.
The potentially most vulnerable unbroken as well as broken stalactites were measured in a pilot cave (Milandre, Switzerland). Four classes of stalactites were defined, according to their shapes. For each of these classes, a vulnerability curve (probability of breaking as a function of peak ground acceleration) was obtained by means of a Monte Carlo simulation. Dynamic amplification as well as heterogeneity of bending resistance within each speleothem were taken into account. Finally, an original statistical approach, valid for incomplete and imprecise data, was developed. This approach allowed to estimate the probability that at least one moderate earthquake has occurred in the past.
I apologise for the length of this ANDYSEZ - may as well go out with a bang! - but the question of speleothem damage, earthquake effects and so on in caves is a fairly common one.
My research is almost as certainly not as detailed as might be needed. So if anyone else wants to chase up this subject there is infinity of sites and authorities to be consulted.
References
Plimer, I 1989 How, When, Where and Why and Earthquake Occurs, Newcastle Herald 30 December 1989, p11
Lacave, C, Koller MG and Levret A, 2000 Measurements of natural frequencies and damping of speleothems, 12th World Conference on Earthquake Engineering, Auckland
Lacave, C, Egozcue, JJ and Koller, MG 2002 Can broken - and unbroken - speleothems tell us something about seismic history?, Proceedings of the 12th European Conference on Earthquake Engineering, London, Paper No. 349
Lacave, C, Koller, MG and Egozcue, JJ 2004 What can be concluded about seismic history from broken and unbroken speleothems, Journal of Earthquake Engineering, 8(3)431-455
Trewby, Mary 2004 Beachcomber: A New Zealand and Pacific Island Miscellany, Random House, New Zealand
The full (pdf) copies of the Lacave et al papers can be found at: <http://www.resonance.ch/pages/communications.asp>