Recent changes in the local hydrology, Lobster Rivulet catchment, Mole Creek karst: The landslides of January 2011
PhD candidate, School of Chemistry, University of Tasmania
Email: debhunter8@bigpond.com
Abstract
Northern Tasmania’s wet conditions of the 2010/2011 summer season commenced in spring, following drought. Caves of the Mole/Lobster drainage sub-system experienced a series of floods. Saturated conditions were maintained in the catchment until 5 mass movements occurred concurrently on the 14th January, and a further 4 in the following days. Included was a 2.5 km debris flow that has changed the hydrology and precipitated some amusing local media coverage. The discussion includes a briefing on local fluviokarst hydrogeology and a destroyed convict built water scheme. Implications for the farming community and the popular wild caves of the sub-system are considered in the context of climate change.
Introduction
The Mole Creek karst, in northern Tasmania, is so-named after the behaviour of one of its streams, which appears from the mouth of Wet Cave only to plunge underground once more after a short distance into Honeycomb Cave. It is a large, densely cavernous fluviokarst, in that much of its catchment is on adjacent non-cavernous rocks. Gordon limestone outcrops extend approximately 10 km (north-south) by 26 km (east-west), between approximately 200 and 600 m elevation (Kiernan, 1989, 1995). Karst drainage is well developed, and surface water is scarce. The karst is bounded to the south by the Great Western Tiers, the escarpment of the Central Plateau horst, rising 1000 m above the Mole Creek valley floor. The “Tiers” name reflects the stepped profile, so formed because of the differential erosion resistance of strata of the Parmeener Supergroup sedimentary rock sequence. Harder, igneous Jurassic dolerite rock caps the Central Plateau, forming columnar cliffs atop the Tiers escarpment, a feature distinctive of many Tasmanian mountains. The Tiers, up to 1320 m in elevation, represent a substantial catchment for the karst. The Lobster Rivulet is one of several sub-catchments draining the Tiers and contributing to the karst drainage system. The Lobster’s hydrology interacts in a complex manner with that of the adjacent sub-catchment of the Mole Creek itself, according to seasonal and flow stage conditions (Kiernan, 1995; Hunter et al, 2008).
Northern Tasmania has a Mediterranean, seasonal distribution of rainfall, annual precipitation in the Mole Creek area ranging from 1111 mm in the valley to >1600 mm in the upper catchments, due to orographic effects (Bureau of Meteorology, 2007).
Like elsewhere in south-east Australia, the Mole Creek area is subject to cycles of drought and flood, due to such influences as the El Nino Southern Oscillation (ENSO). However, the dramatic summer storms on the Tiers that triggered the mass movements of January 2011 in the Lobster catchment are more common occurrences of the north-eastern Tasmania highlands (Langford, 1965). According to locals, supported by the survival of a convict-built water scheme until January, the episode of mass movements is unprecedented in living memory or oral history. The unusual meteoric conditions that led to these events are consistent with the effects of anthropogenic climate change.
Scars in the slope sediments of the Great Western Tiers reveal a history of landslides, with 39 apparent from 2007 mapping (Mineral Resources Tasmania, 2007). However, such events can be infrequent. Two residents of Caveside, in the Lobster Rivulet catchment, recall two narrow landslides occurring more than 50 years ago. Those scars are now difficult to pick out under the (recovered) forest canopy. The escarpment of the Tiers is slowly retreating by weathering of the softer sedimentary rocks underlying the dolerite cap; the dolerite columns topple down as support is removed. Glacio-fluvial and peri-glacial processes have eroded the weathering products downslope. These sediments have formed mantles over the karst geological contact and fans at the footslopes of the escarpment (Kiernan, 1995). The slope deposits have stabilised under a cover of wet sclerophyll (mixed) forest established since the Holocene optimum climatic period. Where slope deposits mantle the karst contact, they function as a perched aquifer, gradually releasing water through constricted vadose conduits in the epikarst to maintain water flow in the karst systems through dry seasons. However, there are additionally a small number of direct stream inflows, or cave swallets.
Media reports covered extensive, record-breaking floods in northern and north-eastern Tasmania over the summer of 2010-11. At Caveside on 14th January 2011, a local farming couple were having morning tea when they heard the Westmorland debris flow descend the mountainside; and when they looked out of their window, they saw tonnes of silt and gravel had swept out of the bush onto their paddock (Figure 1). A stream of water and silt continued from the toe of the debris flow, sustaining an overland water flow for four days. Like other locals in a series of interviews on local radio (ABC 7NT, Country Hour, 24th January 2011), they simply expressed their amazement and privilege, in spite of losses to property and livestock, to have witnessed such amazing events in their lifetime.
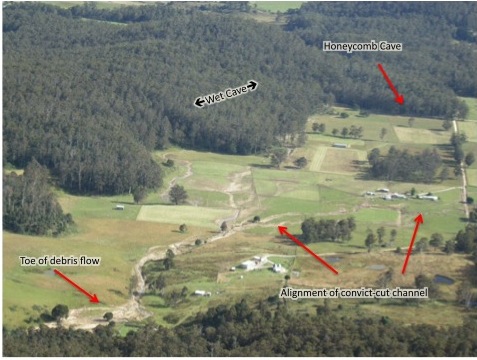
Figure 1: Oblique aerial photograph (looking north from the Tiers) taken 10 days after thedebris flow. Paths of silt show the distributory flow of water from the toe of the slide, which occurredbecause the carrying capacity of the depicted convict-cut channel was exceeded. Where this artificialchannel passes under the road to the right, overflow also followed the road, causing substantial damage.
A series of floods over spring and summer followed El Nino drought conditions that had prevailed for three years. Finally, a series of storms which brought 195.5 mm of rain over five days at Caveside (unpublished weather diary of the author) destabilised the mountainside, already saturated by the summer season’s La Nina conditions. A total of nine landslides became visible on the slopes of the Lobster Rivulet catchment, triggered by these storms. In contrast, no landslides were apparent in adjacent catchments. Summer storm cells can have localised effects. The mass movements include a slide approximately 600 m in length on the eastern flank of the Lobster Rivulet, its toe resting in the Rivulet gorge. Although not as obvious as this example when viewed from the valley floor, the Westmorland debris flow is by far the most extensive of the features at over 2 km in length, and is the most important in terms of hydrological change in the catchment. The landslides are depicted in context with karst drainage in Figure 2.
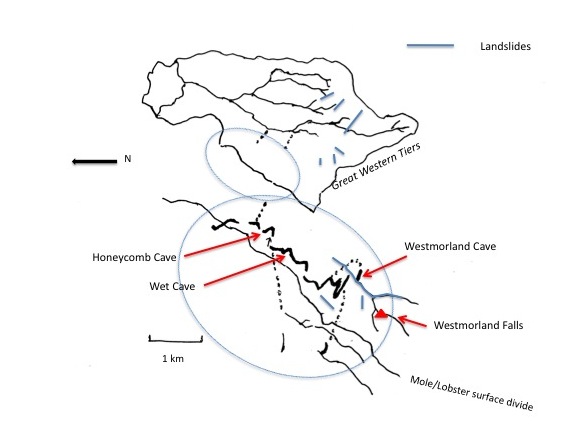
Figure 2: Mass movements in the Lobster Rivulet catchment, January 2011 (drawn after Hunter et al., 2008).The path of the debris flow past Westmorland Cave has scoured a previous overflow channel into asubstantial surface watercourse. The inset shows the Lobster/Mole master cave system; the surfacedivide shown is double-breached by the drainage. Solid lines show known caves. Dotted lines showsimplified (proven and inferred) karst hydrological relationship of the Lobster and Mole karst sub-systems (after Kiernan, 1989).
From Figure 2, the path of the Westmorland debris flow bypasses the once-spacious cave swallet, where it then follows the alignment of a vehicular track, constructed to enable access for maintenance and improvement of a historic water diversion at the cave. The track provided a straight path of least resistance for the debris flow to continue to the farmland at the footslopes of the escarpment, where the slope gradient reduces. Most of the water that used to flow into Westmorland Cave now flows down the scour gully of the debris flow, past a blockage of broken trees and boulders at the cave’s inflow entrance. Only a trickle of water now passes under that blockage into the cave. Prior to the mass movement, the small water scheme, originally built by convicts, channelled some of the flow down a natural overflow channel to ensure an all-season flow of water, thence reticulated in a ditch through the farms downstream. The convicts cut a 3 x 3 x 3 foot channel (“the nine-foot”) through a limestone wall immediately upstream of the cave inflow, and a log was felled to act as a dam that diverted the stream at low flow stage into the cutting and hence into the overflow channel. At high flow stage, water flowed over the log and into a smaller adjacent entrance customarily used by cavers, and the swallet still performed in a manner close to its natural hydrological function. The cave would have first filled to capacity during a flood pulse, the overflow channel only operating if that capacity was exceeded. Problematically for local pastoralists and their infrastructure, the capacity of the blocked cave to provide a buffer against potentially damaging flood pulses is now seriously compromised, and the majority of the stream now flows down the scour channel and hence into the artificial ditch (Figure 3).
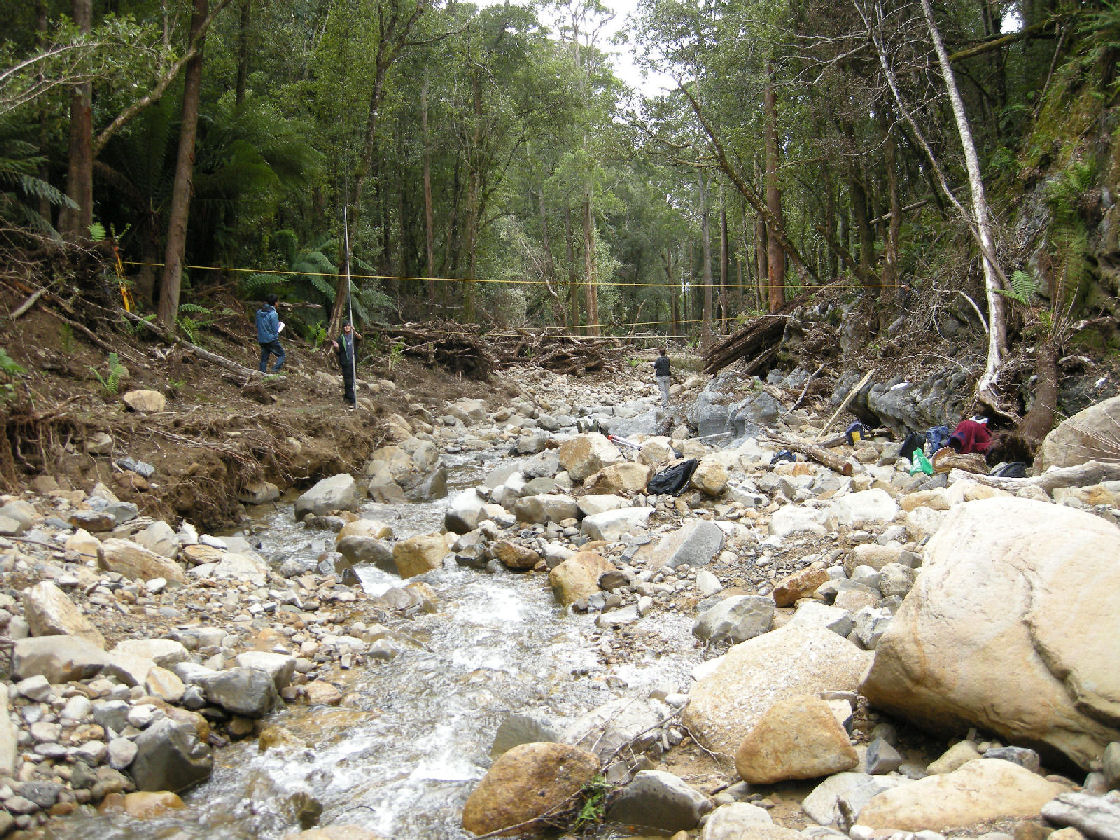
Figure 3: Scour gully looking downstream from the vicinity of Westmorland Cave entrance.Note for scale the people making measurements (geomorphology class from the University of Tasmania).From evidence of the removal of soil, the scour was 19.3 m wide at the foreground survey cross-section.The scene in this picture was previously fully covered by the forest canopy, trees growing up to thebank of the small overflow channel.
Debris flows are catastrophic (sudden) mass movements, where saturated slope materials move as if the total was a liquefied mass. At Westmorland, the tributary stream from Westmorland Falls a short distance upstream of the cave (Figure 1) increased the volume of water and the energy of the mass movement in the vicinity of the cave. Trees and soils in the path of the debris flow were carried along to join the mass. Larger boulders, rocks and broken trees deposited first, followed by gravels and sands further downslope, as the energy of the flow lessened with the slope gradient. Silt-laden waters then dispersed in a distributory manner over the glacio-fluvial fan at the footslopes in the flooding conditions, causing flooding on the farms and passing overland to cause washouts at entrances to Honeycomb Cave (Figure 1). Within the first few days of the debris flow, council workers had deepened the ditch through the farms to increase its capacity and repaired the washed-out road. Vehicular access being impossible, the farmers moved rocks by hand at the cave mouth to direct water flow into the cave mouth concealed beneath the debris (Figure 4).
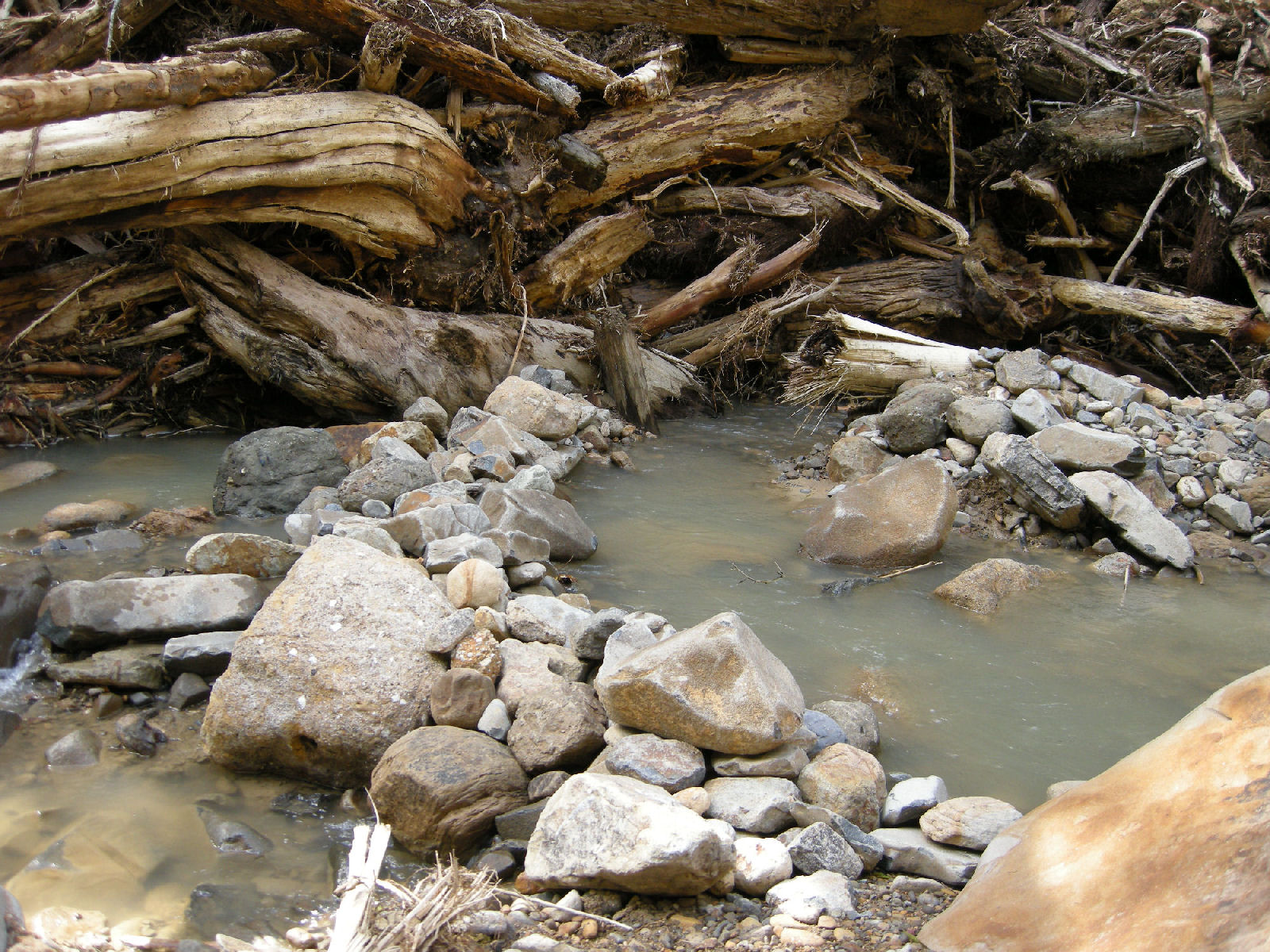
Figure 4: Blockage of Westmorland Cave. To help protect their farms downstream from more floods like those of January 2011,in the days following the debris flow, local farmers diverted as much of the creek as they could backtoward the cave swallet under the blockage at its entrance. Note the mixture of rounded, reworkedrocks from the streambed, and angular rocks more recently dislodged from their origins.
An attempted inspection of the entrance series of Westmorland Cave from the inside in May 2011, accessed by way of a vertical entrance to a large chamber, revealed tree fern root mats and broken woody debris to 30 cm diameter blocking the inflow passage where it narrows before the large chamber. Below that chamber, the deposition of two sequences of sand and fine organic matter in a depression in the stream bed and the lack of new large woody debris attest to the efficiency of the blockage at the entrance rift and indicate the possibility of sediments constricting drainage in the cave. An old photograph shows evidence of high-energy flows in the past, with sandstone boulders from hundreds of metres above the cave lodged in the initial entrance rift (Figure 5).
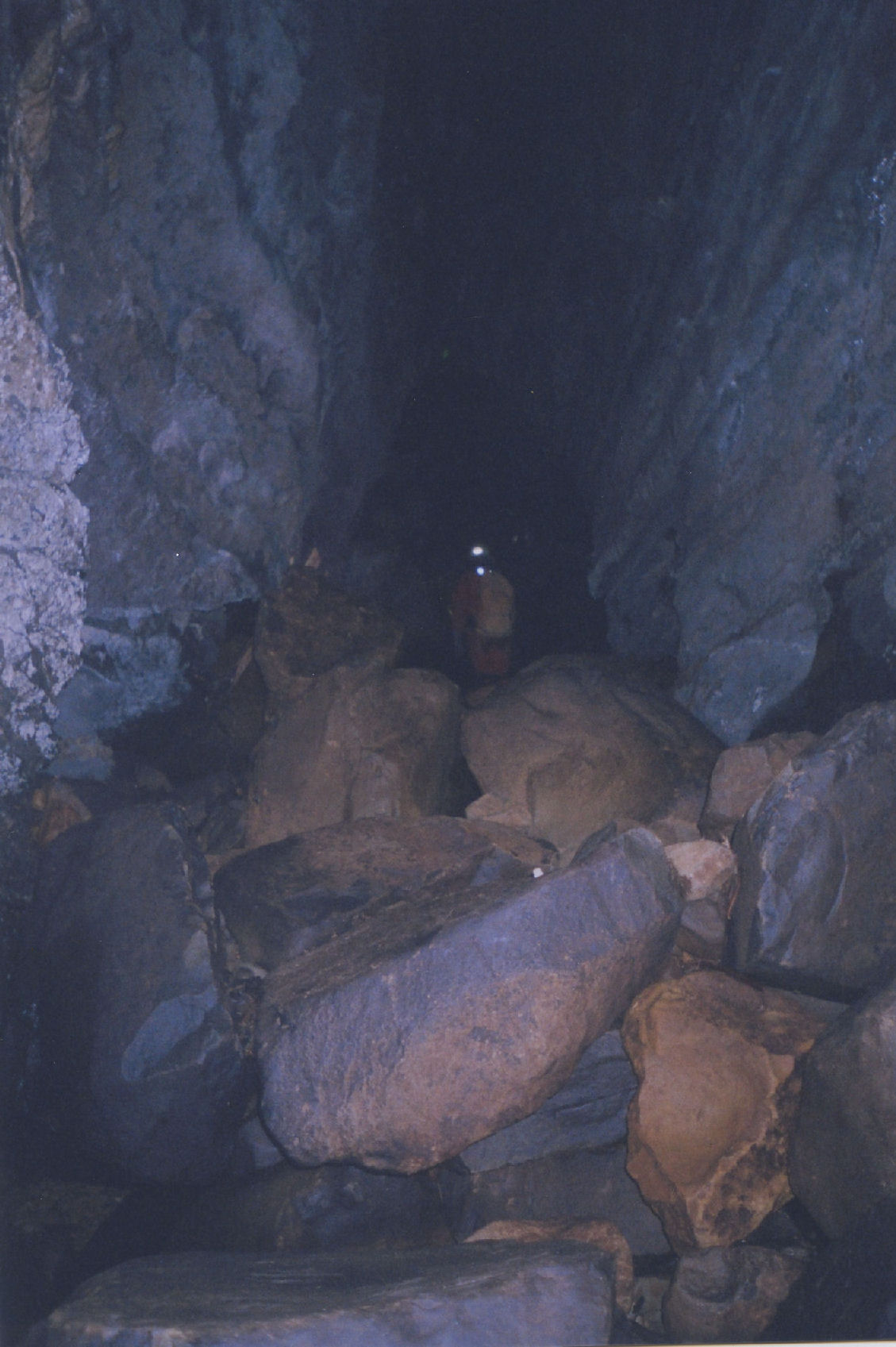
Figure 5: Caver climbing out the entrance rift of Westmorland Cave prior to its recent blockage.Large sandstone boulders can be seen. Further into the cave, the entrance passage narrows, where rootmats and woody debris now block it.
Previously, over more than 30 years experience, I had only ever known of flash flooding in Honeycomb Cave. However, while the overland flow persisted for four days, water submerged even high-level passages that I had never known active during flash floods. As the water subsided, sights to be seen included tonnes of displaced glacial gravel deposits and shifted log-jams. Since then up to the time of writing in June 2011, regular visits to the cave have revealed that the numbers of cave-adapted fauna have crashed compared to prior to the flooding.
Conclusion
It is quite possible that such an event as the debris flow in January 2011 has happened before at this site. However, enough time had passed since any previous similar event, that the boulders in Figure 5 were firmly lodged in their places over the long-term, resisting the force of historic flooding water flows, and a closed forest canopy overhung the 2 m wide overflow creek-bed, with riparian vegetation growing on banks with well-structured soils. Few pieces of large woody debris had previously been known in Westmorland Cave, and were confined to a long, tall rift section beyond the entrance passage and a large daylight hole chamber. However, organic matter on the ceiling from water backing up into the large chamber had been noted in the past. Westmorland Cave’s hydrological function is likely to be compromised compared to its previous function, due to the limited transmissivity of the phreatic level passage/s, some 600 m beyond the entrances, and the possibility of siltation in those passages. While there may once have been a wetland there absorbing cave overflow water, it has been cleared for farming, a land use with infrastructure requiring protection from further catastrophe. The cave itself may find numbers of curious cavers negotiating the vertical entrance, compromising the delicate organic-rich earth slope under the daylight hole that supports the cave’s diverse ecosystem.
Honeycomb Cave may react differently to flood events than in the past. Being the most important recreational wild cave at Mole Creek, this has implications for caver safety and further erosion. While erosion stabilisation works are progressing at the cave, further floods could hamper long-term stability.
The mass movements of January 2011 could represent the vanguard of geomorphic change brought about by anthropogenic climate change. The local community has exhibited adaptability in repair of infrastructure and cave entrances, the efficacy of which remains to be tested by the next series of extreme meteoric conditions.
If indeed climate change means more frequent and more extreme weather events, an increase in erosion rates of the slope deposits on the Great Western Tiers escarpment above the karst could reduce the hydrologic storage and release function of perched epikarst aquifers, causing higher flows in wet times and depriving cave ecosystems of flow in dry periods. Many in the farming community could find their water supplies affected. Should further hydrological change continue, the proportion of Westmorland’s water finding its way to each of the two karst systems, Mole and Lobster, could change.
References
Bureau of Meteorology. (2007). Archival climatic data provided by facsimile, Bureau of Meteorology, Launceston.
Hunter, D., Lewis, T. and Ellis, J. (2008). Karst drainage relations with catchment land use change, Mole Creek, Tasmania, Australia. Cave and Karst Science 35 (3).
Kiernan, K. (1989). Karst, Caves and Management at Mole Creek, Tasmania. Occasional Paper No. 22, Department of Parks, Wildlife and Heritage, Hobart.
Kiernan, K. (1995). An Atlas of Tasmanian Karst. Research Report No. 10, Tasmanian Forest Research Council, Inc., Hobart.
Langford, J. (1965). Weather and Climate. In Davies, J.L. (ed) 1965: Atlas of Tasmania. Lands and Surveys Department, Hobart.
Mineral Resources Tasmania. (2007). Great Western Tiers landslide data summary and mapping provided by Mineral Resources Tasmania, Hobart.