CHROMATED COPPER ARSENATE TREATED WOOD IN CAVES
1Swabey Consulting, 4 Allans Beach Road, Portobello, Dunedin, New Zealand
+64 3 478 0909. stephen@peninsula.co.nz
2Department of Geography, University of Auckland, Private Bag 92019, Auckland, New Zealand
ABSTRACT
The environmental impact of chromated copper arsenate (CCA) treated wood in caves is examined using two complementary approaches. Laboratory studies of leaching indicate rates are lower in karst waters for both arsenic and copper. Leaching rates are high immediately following timber treatment, declining rapidly. In nine weeks, 16%± 7% of the arsenic and 2% of the copper leached from hazard-class 3 (H3) wood, whilst 2% of the arsenic and 3% of the copper leached from H5 wood in the same period.
Arsenic leaches to a greater degree from H3 wood than H5 wood, but copper leaches less from H3 wood than H5 wood. Field studies of CCA treated structures in caves revealed arsenic and copper concentrations in standing water that would be toxic to some freshwater crustaceans and algae respectively. 1.2% of the arsenic and 1% of the copper leach per annum from a two-year old treated cave weir.
CAPSULE
This study demonstrates that leaching rates of arsenic and copper from chromated copper arsenate treated wood in caves are lower than in non-cave environments, but both metals may reach toxic concentrations in certain conditions in caves.
INTRODUCTION
Wood used in structures exposed to water is susceptible to rot, typically caused by fungus growth. Although some hardwoods are more resistant to fungal attack than softer woods, the limited availability of the former species makes preservative treatment of susceptible wood a more economically viable solution. A number of preservative treatments have been developed to extend the life of wood in wet environments.
Globally, the most common wood treatment process is the introduction of chromated copper arsenate (CCA) to the wood under pressure. Alternative preservatives exist, where arsenic has been replaced with phosphate (CCP), boron (CCB) or fluoride (CCF) and ammoniacal copper quaternary (ACQ) treatment is also used. Organic solvent-based preservatives are available, but their cost prohibits use in large-scale applications (Hedley, 1997). CCA treated wood is used extensively as a building material, in marine structures such as piers, and for fencing.
A potential problem with the use of treatment preservatives like CCA is leaching of the component chemicals to the surrounding environment. Although treatment methods are intended to 'fix' the components in the wood, they may leach if the wood is used before the chemicals have fixed. The fixing reaction may require two weeks to complete in freshly-treated wood in temperate environments in summer. Cooler temperatures in winter retard the reaction and weeks or months may elapse before the reaction completes (Hedley, 1997).
Hazard class | Intended use | Hazard |
H1 | Inside, protected from weather | Insect borers |
H2 | Inside, protected from weather | Insect borers and termites |
H3 | Outside, above ground | Insect borers, termites and decay |
H4 | Outside, in ground contact | Insect borers, termites and severe decay |
H5 | Outside, in ground, critical situations | Insect borers, termites and very severe decay |
H6 | Marine environments | Marine organisms |
Table 1. Hazard classes, their intended use and the expected hazard (Hedley, 1997).
H3 | H4 | H5 | |
Retention (mass/mass) | 0.37% | 0.72% | 0.95% |
Proportion As | 0.11% | 0.22% | 0.29% |
Proportion Cu | 0.08% | 0.16% | 0.22% |
Proportion Cr | 0.16% | 0.31% | 0.41% |
As in each block | 0.0087g | 0.0174g | 0.0237g |
Cu in each block | 0.0064g | 0.0128g | 0.0180g |
Cr in each block | 0.0127g | 0.0258g | 0.0334g |
Table 2. Retention (% mass/mass and grams) of concentrate and individual component metals in wood treated to the three hazard class standards investigated. Retention percentages are those suggested in Koppers Arch treatment regimes. Average mass of metals in each block is calculated from the initial weight of the block and suggested retention rates.
A number of laboratory and field studies have examined the leaching of the individual components from treated wood in marine and freshwater environments. The effect of the component chemicals in CCA treated wood on non-target species has been examined in a number of studies. The acute and chronic toxicity of leachate from CCA treated wood in marine environments is equivocal (Brown and Eaton, 2001, Weis et al, 1992). CCA leachate affects biota quantity and diversity in short term (daily and monthly leachate) experiments (Weis and Weis, 1996b), but experiments over an 18 month period show no effect on species diversity (Brown and Eaton, 2001). High, but declining initial levels of leachate when wood is first exposed to the environment may partly account for this pattern. The large volume of diluting water available in marine environments partially explains the low impact of leachate in later periods (Weis and Weis, 1996a).
Factors affecting leaching rates include salinity of the leaching solution, pH, temperature and the proportion of end grain exposed (Hingston et al, 2001). Copper and chromium leaching rates increase as conductivity and salt content of water increase. Higher rates of leaching occur at higher pH, probably because additional H+ ions are available for acid-ion-exchange reactions. Increasing temperature also increases leaching rates in most studies. Leaching from the end grain is up to forty times greater than leaching from the lateral grain (Hingston et al, 2001).
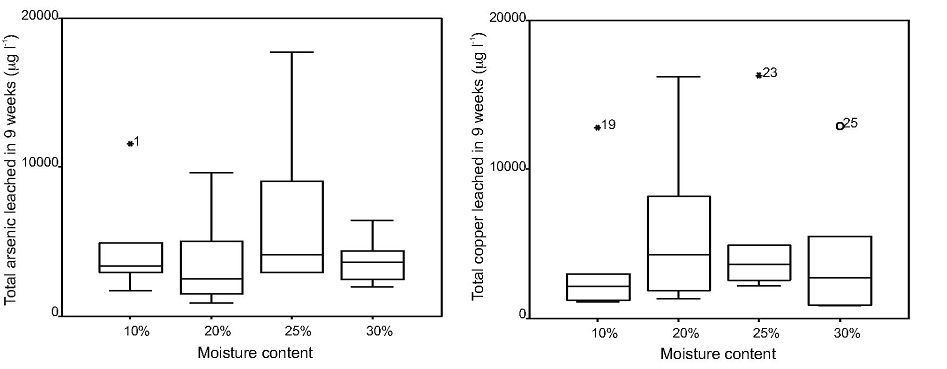
Figure 1. Arsenic and copper leached from treated wood blocks of varying moisture content in nine weeks.
ARSENIC
Environmental sources of arsenic include natural sediments, geothermal waters (Takamatsu et al, 1982), atmospheric pollution from smelting and other industrial processes (Ondov et al, 1996), agricultural runoff and disposal of industrial (Erry et al, 2000) and agricultural wastes. Background concentrations of arsenic in streams may vary from 0.1-1.7 μg l-1 (ppb). Arsenic may be found in groundwater in a wider range, from 0.01-800 μg l-1 (Matschullat, 2000). Arsenic can be present at very high levels in phosphate detergents, fertilisers and pesticides (Huang and Liaw, 1978). For example, soils in Missouri previously treated with the pesticide lead arsenate contained as much as 300 ppm total arsenic (Hess and Blanchar, 1977). Soils in fields irrigated with water polluted by mining waste and geothermal water in Japan showed total arsenic concentrations up to 367 mg l-1 (Takamatsu et al, 1982).
Arsenic is present in toxic concentrations in aquatic ecosystems and in water consumed by non-aquatic organisms. The inorganic As (V) form is thought to be more prevalent, but less toxic than the As (III) form (Hingston et al, 2001). The toxicity of trivalent As to freshwater organisms ranges from a lethal concentration killing 50% of specimens (LC50) at 96 hours of 0.2 mg l-1 for a freshwater epigean crustacean, Gammarus fossarum to a 96 hour LC50 of 127.4 mg l-1 for the red worm Tubifex tubifex (Canivet et al, 2001). The effect concentration (EC50) for unspecified algae in Massachusets ponds ranges from 22 μg l-1 to 72.5 mg l-1 (Knauer et al, 1999). Arsenic reduced to arsenite may restrict rice productivity in areas affected by high-arsenic mining waste or geothermal waters (Takamatsu et al, 1982). Arsenic probably bioaccumulates from vegetation (Bennett et al, 2000) to herbivores (Erry et al, 2000, Weis and Weis, 1992) and carnivores (Mason et al, 2000, Weis and Weis, 1999), although other work has shown no trophic transfer of metals from CCA wood (Adler Ivanbrook and Breslin, 1999).
COPPER
Copper occurs naturally as a metal ore. It may be concentrated in the environment by human activities such as mining, but is also used in wire, electrical and electronic equipment, brass, electroplating, construction and as copper sulphate in agriculture (Bodek et al, 1998). The background concentration of copper in cave stream water has previously been reported as 8 μg l-1 at a single site (Dickson et al, 1979). Copper is the most bioconcentrated of the three metals in CCA treatment (Weis and Weis, 1999) and may have a higher toxicity than arsenic, with the metal toxicity rank being Hg > Cd > Cu > Cn > Ag > Zn > Ni > As > Cr > Pb in the mysid Mysidopsis bahia (Lussier et al, 1985). Copper has a wide range of toxicity in various environments. Freshwater Chlorella algae show a 72 hour EC50 of 1.5 μg l-1 in low CaCO3 waters at pH 6.5 (Franklin et al, 2000). The freshwater daphnid Daphnia magna has a 48 hour LC50 of 18.9 μg l-1 Cu from CuSO4 in a pH range of 6.5-8.2 (Martin and Rodgers, 2000). Embryos of the amphibian Bufo arenarum exhibit a twenty-four hour LC50 of 85 μg l-1 (Herkovits and Helguero, 1998).
Sample | Description | As (μg l-1) | Cu (μg l-1) |
10 | Ruakuri Cave - drips from inlet high on cave wall | 0.00 | 1.41 |
11 | Ruakuri Cave - water from pool under boardwalk below 10 | 273.59 | 3.66 |
24 | Ruakuri Cave, Holden's Cavern - water flowing on boardwalk | 1.63 | 1.37 |
28 | Ruakuri Cave, Ghost Walk - water dripping from boardwalk, high flow rate | 5.02 | 0.94 |
30 | Mangawhitikau Cave - stream downstream of weir | 0.57 | 1.19 |
M | Mangawhitikau stream upstream of cave entrance | 0.00 | 0.84 ± 0.13 |
36 | Glowworm Cave - drips onto wood pile | 0.00 | 1.55 |
Table 3: Water samples obtained from caves in Waitomo.
Arsenic parameters | Copper parameters | |||||||
Furnace step | Temp (°C) | Ramp time (s) | Hold time (s) | Gas | Temp (°C) | Ramp time (s) | Hold time (s) | Gas |
1 - drying | 80 | 5 | 1 | Inert | 90 | 10 | 5 | Inert |
2 - drying | 140 | 30 | 10 | Inert | 120 | 30 | 10 | Inert |
3 - ashing | 800 | 15 | 5 | Inert | 800 | 15 | 5 | Inert |
4 - gas clear | 800 | 0 | 1 | None | 800 | 0 | 1 | None |
5 - atomisation | 2500 | 2 | 0.5 | None | 2300 | 2 | 0.5 | None |
6 - tube clean | 2600 | 1 | 0 | Inert | 2400 | 1 | 0 | Inert |
Table 4. Graphite furnace parameters used.
Arsenic | Copper | |||||||
Period | Water | n | Mean | Std dev. | Std Error Mean | Mean | Std dev. | Std Error Mean |
one hour | Distilled | 11 | 50.8 | 37.9 | 11.4 | 143.5 | 139.1 | 44.0 |
Karst | 12 | 53.9 | 54.7 | 15.8 | 113.8 | 87.3 | 25.2 | |
twenty-four hours | Distilled | 12 | 391.3 | 148.7 | 42.9 | 551.9 | 622.7 | 196.9 |
Karst | 12 | 237.2 | 89.2 | 25.7 | 206.8 | 266.9 | 80.5 | |
four days | Distilled | 12 | 987.8 | 533.6 | 154.0 | 817.3 | 887.9 | 267.7 |
Karst | 12 | 732.5 | 346.1 | 99.9 | 636.4 | 564.7 | 163.0 | |
two weeks | Distilled | 12 | 1379.9 | 1193.5 | 344.5 | 3187.3 | 3052.2 | 881.1 |
Karst | 12 | 1493.3 | 1439.9 | 415.4 | 573.6 | 385.2 | 111.2 | |
nine weeks | Distilled | 12 | 6280.9 | 4578.6 | 1321.7 | 3090.5 | 1732.1 | 500.0 |
Karst | 12 | 3145.8 | 2124.3 | 613.2 | 674.6 | 649.2 | 187.4 |
Table 5. Concentration of arsenic and copper in 200 ml of distilled water and karst water in which various CCA treated wood blocks were placed. Waters were completely replaced at the end of each period. All values in μg l-1.
Various environmental factors affect copper toxicity. The coastal mysid Praunus flexuosus has a 96 hour LC50 of 30.8 μg l-1 in summer, but winter toxicity of copper to P. flexuosus is insignificant, probably because this species has low physiological activity during this season (Garnacho et al, 2000). Mortality in the catfish Ictalurus punctatus when exposed to 1.25 mg l-1 CuSO4 decreases from 90% at calcium hardness of 10 mg l-1 CaCO3 to 5% at 400 mg l-1 CaCO3 (Perschbacher and Wurts, 1999). Lower pH conditions may increase copper toxicity (Franklin et al, 2000), although the opposite effect is also reported (Meador, 1991). Copper bioaccumulates in the troglobitic (obligatory cave-dwelling) crayfish Orconectes australis australis at higher levels than in the troglophilic (facultative cave-dwelling) crayfish Cambarus tenebrosus, possibly because of increased longevity of the former (Dickson et al, 1979).
CCA treated wood is used in a number of caves in New Zealand, the United States of America and other countries of the world, because it offers a cheap, rot-resistant construction material for typically wet conditions. However, the environmental impact of CCA treated wood in caves has never been assessed. Cave organisms are usually highly adapted to cave conditions, tolerating a restricted range of temperature, humidity and food levels. Adaptations to these low energy conditions may increase longevity - troglobitic crayfish may live as long as 100 years (Dickson et al, 1979). Organisms in isolated cave systems cannot readily move to alternative habitats, so arsenic and copper leached from local CCA treated wood may have more significant impacts. This work examines leaching rates of arsenic and copper from CCA treated wood in temperate caves from two perspectives. The first experiment compares leaching rates in water obtained from a karst setting and in distilled water. The second experiment examines the magnitude of leaching in selected caves in New Zealand.
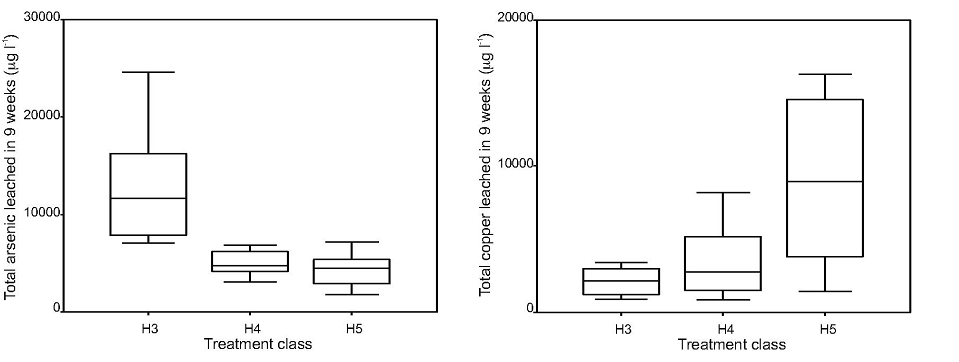
Figure 2. Arsenic and copper leached from treated wood blocks in nine weeks.
MATERIALS AND METHODS
Several experiments were designed to investigate a number of hypotheses - that arsenic and copper:
- Leach to the same degree from wood with different initial moisture contents
- Leach to the same degree from wood treated to different hazard class standards
- Leach to the same degree from wood placed in either distilled or karst waters
- Leach at the same rate from treated wood of various ages
- No longer leach from CCA treated wood that has been in a cave for a long period of time
- Are not detectable in water running over CCA treated wood in a cave
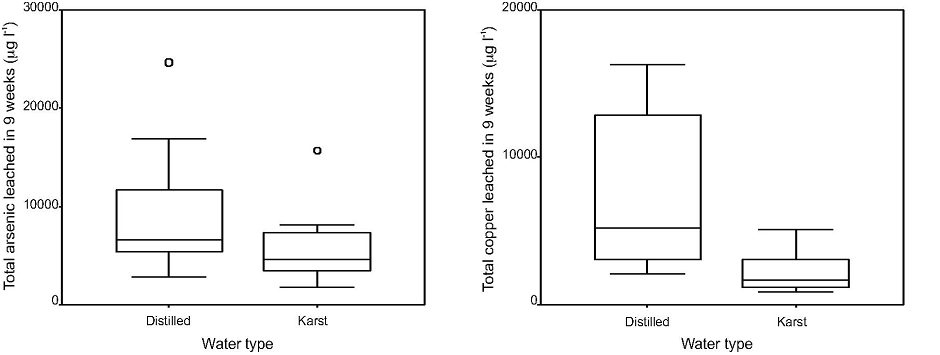
Figure 3. Total arsenic and copper leached from treated wood blocks in distilled water and karst water in nine weeks.
Wood is treated with CCA to reach one of several 'hazard class' standards (table 1). The amount of arsenic, chromium and copper introduced to the wood depends on the hazard class required (table 2). The preservative penetrates to different depths in heart wood and sap wood, because of the differing densities. To avoid variation in leaching rates caused by variation in wood type, this experiment uses wood blocks obtained from one source and treated immediately prior to analysis.
Untreated pine wood with a range of moisture contents was obtained from Cashmore Brothers Limited sawmill, Silverdale, New Zealand. Kiln dried wood with a nominal 10% moisture content was obtained and wood with nominal moisture content of 20%, 25% and 30% was selected from the sawmill yard. Actual moisture levels were determined as 12%, 20%, 25% and 34% respectively by gravimetric methods. The wood samples were cut into a standard block size of 15 x 25 x 50 mm as prescribed by ENV. 1250:2 (BSL, 1994). Four blocks of each type were packaged in open mesh material for each treatment. CCA treatment to hazard classes H3, H4 and H5 was undertaken on wood with the four different moisture levels. Cashmore's own plant performed the H3 and H4 treatment and Kumeu Timber Treatments Limited, Kumeu, New Zealand performed the H5 treatment.
Both treatment companies treated the timber with Koppers Arch Tanalith CCA preservative. Tanalith CCA is supplied as a stock solution with salt as 60% weight by volume (w/v) and oxide as 103.2% w/v. The same preservative concentrate is used to treat wood for all three hazard classes examined here. Treatment to the various hazard classes is defined by the proportion of preservative retained in the timber by mass (table 2). The solution is diluted with water before application to timber under pressure. The proportions of the individual metals in each treatment solution remain the same, but the actual quantities vary depending on the hazard class treatment required.
A total of 64 blocks were used - two blocks for each treatment process and moisture content level in each water type, and one pair of controls for each situation. For each hazard class and moisture content level, one pair of blocks were leached in distilled water and the other pair in water obtained from Mangawhitikau cave stream. The Mangawhitikau stream is a sinking stream in the Te Kuiti district, North Island, New Zealand that flows over limestone for several kilometres upstream of the sampling point. The CaCO3 content of the Mangawhitikau water was 80 mg l-1.
Treated wood of various ages was obtained from the Cashmore Brothers storage yard. The time elapsed since treatment was estimated at 1 week to over 3 years.
These woods had been treated to a variety of hazard class standards. An untreated control sample was also obtained, with an age of 4 months. These samples were also cut to the BSI standard size and then placed in 200 ml of distilled water.
CCA treated wood was obtained from four show caves at Waitomo. Three of these caves - Waitomo Glowworm Cave, Aranui Cave and Ruakuri Cave - were previously operated as tourist attractions by the Tourist Hotel Corporation, New Zealand.
All three caves had treated timber and hardwoods installed for cave structures in the 1960s and 1970s. Some timber in the more heavily visited Glowworm Cave was replaced in the mid-1980s and further replacement took place during 2001 (Robert Tahi, Glowworm Cave Manager, personal communication, 2002).
Nine samples of wood was obtained from boardwalks and handrails in the Glowworm Cave - from both wet and dry situations. Seven samples were obtained from boardwalk that had recently been removed from the cave.
Fifteen samples were obtained from Ruakuri Cave boardwalks and handrails. This cave has not operated as a show cave since 1987 and the structures are currently being replaced with stainless steel.
Four wood samples were obtained from boardwalk in the Aranui show cave and two samples from a weir and jetty installation in the Mangawhitikau show cave. Wood in Aranui Cave also dates from the 1960s or 1970s, whilst wood in the Mangawhitikau cave was installed in November 1999.
Six water samples were obtained from the caves (table 3), including drips from cave inlets, water running over treated timber boardwalks, standing water in which treated timber was sitting and natural cave waters.
Wood samples obtained from the caves and from the wood processing plants were leached in 200ml of Mangawhitikau or 200ml of distilled water in 250ml quartz glass beakers, rather than the BSI standard of 500ml (BSI, 1994). The beakers were covered to limit evaporation. Samples for analysis were obtained at one hour, twenty-four hours, four days, two weeks and nine weeks. The leaching water was replaced completely when a sample was obtained for analysis.
Chemical preparation of the water samples for arsenic determination was by placing 50ml of sample in a 250ml beaker with 1 ml 30% hydrogen peroxide and 1 ml concentrated nitric acid. This solution was heated at 95°C for 2 hours and then transferred to a volumetric flask and diluted to 50ml with distilled water.
The analyses were performed on a GBC Avanta M atomic absorption spectrophotometer with a graphite furnace and PAL3000 automated sampler. A modifier of 10 L of 0.2% nickel nitrate was added to each sample prior to analysis by the instrument. The arsenic lamp was operated at a wavelength of 193.7 nm and a current of 8.0A. The furnace parameters for arsenic determinations are given in table 4.
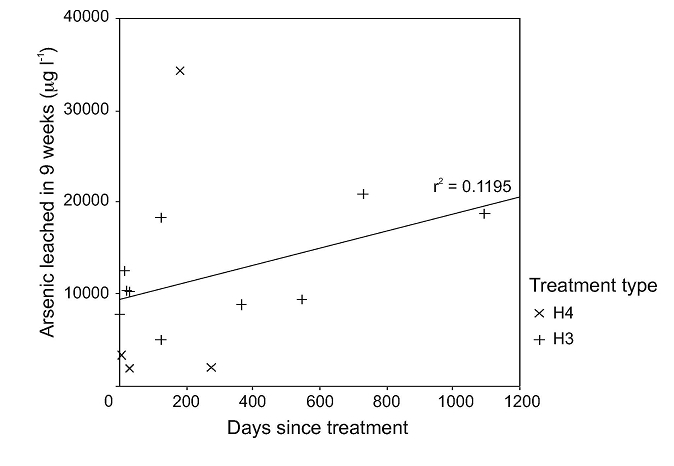
Figure 4. Arsenic leached from treated wood blocks of varying ages in nine weeks. Line of best fit is for H3 timber.
RESULTS
The summarised results from the leaching experiments are presented in table 5 with the measured concentration. Note that this is the concentration at the end of the leaching period, not a pro-rated concentration.
LEACHING FROM WOOD WITH DIFFERENT MOISTURE CONTENT
The range of different moisture content woods does not appear to significantly affect the general leaching rate of either arsenic or copper in either type of water (figure 1).
LEACHING FROM WOOD TREATED TO DIFFERENT HAZARD CLASS STANDARDS
Arsenic leaches most from H3 wood, followed by H4, with H5 timber leaching least. In contrast, copper leaches more from H5 wood, with H4 next and H3 least (figure 2). These distributions generally hold when the data is examined by moisture content, although more copper leaches from 20% and 25% moisture wood than 10% and 30% moisture wood and more arsenic leaches from H5 wood than from H4 wood at 30% moisture.
ARSENIC LEACHING IN DISTILLED AND KARST WATERS
An independent t-test on the mean arsenic levels leached from wood in distilled water and karst water in one hour, twenty-four hour, four day, two week and nine week periods was performed. The t-test indicates significant differences in mean arsenic leaching rates between the two types of water for the twenty-four hour and nine week periods. Although total arsenic leached in nine weeks is not significantly different in bulked analysis of the two waters (i.e. at all moisture levels), there is lower leaching in karst water when considering moisture contents separately. Arsenic leaches most readily in distilled water (figure 3).
COPPER LEACHING IN DISTILLED AND KARST WATERS
An independent t-test for copper leaching in the two waters over different periods shows significant differences between leaching rates in distilled and karst waters at two weeks and nine weeks and also for the total copper leached in nine weeks. There is a also clear difference between total copper leaching in nine weeks from different moisture content woods in the two waters. Copper also leaches most readily in distilled water (figure 3).
ARSENIC AND COPPER LEACHING FROM WOOD OF VARIOUS KNOWN AGES
Leaching in distilled water of copper and arsenic from H3 and H4 wood stored in uncovered timber yards alters through time (figure 4). There is a trend (r2 = 0.12, n=14) for greater rates of arsenic leaching from the H3 wood through time, but a concomitant decline in copper leaching (r2 = 0.04, n=14). The data set for H4 wood is too limited to draw conclusions.
ARSENIC AND COPPER LEACHING FROM WOOD INSTALLED IN CAVES
Arsenic and copper were found to leach from all wood samples obtained from existing cave structures around Waitomo. K-cluster analysis using three clusters suggests arsenic leaching rates are more significant (F ratio of 363) and copper leaching rates less so (F ratio of 15).
Wood from the Glowworm Cave had arsenic/copper leaching rates grouped at three levels. The lowest leaching rate of 4-45/36-94 μg l-1 in nine weeks (5 samples) was from older wood with evidence of significant rot. These timbers are probably hardwoods installed in the 1960s-1970s. The arsenic and copper leaching from these timbers may derive originally from wood installed at a later date. The second group of two samples had low leaching rates of 377/43 and 392/100 μg l-1, and these are probably also timbers installed in the 1960s/1970s. The final group of two samples had high leaching rates of 10556/1037 and 25627/494 μg l-1. These timbers were installed in the cave during the last year.
In Ruakuri Cave, two different rates of arsenic/copper leaching were found. The lowest rates found were 961-4875/134-478 μg l-1, with a higher group leaching at 12262-63260/281-2617 μg l-1. The two samples from Mangawhitikau Cave leached arsenic at 10367 and 14385 μg l-1 and copper at 319 and 423 μg l-1. Aranui Cave samples had arsenic/copper leaching rates of 4462-5839/124-225 μg l-1, suggesting timber from just one source had been used.
ARSENIC AND COPPER IN CAVE WATERS
Arsenic and copper were found in a limited number of the cave water samples obtained. The background level of arsenic entering caves in the Waitomo district through recharge water was 0-1.6 μg l-1. No arsenic was found in the Mangawhitikau Cave stream above the weir, but 0.57 μg l-1 was found below the CCA timber weir, suggesting that arsenic was leaching from the weir. Water that dripped from a CCA treated timber boardwalk in Ruakuri Cave had low levels of arsenic (5.0 μg l-1), whilst water from a pool in which treated timber was soaking had an arsenic concentration of 273.6 μg l-1.
The Mangawhitikau stream has a copper background level of 0.84 ± 0.13 μg l-1, with recharge water in Ruakuri Cave containing 1.41 μg l-1 and in the Glowworm Cave 1.55 μg l-1. Water flowing over CCA timber in the Ruakuri Cave did not have copper concentrations elevated above background, but water from the pool containing CCA timber had copper concentrations of 3.66 μg l-1. Below the weir in Mangawhitikau Cave stream, copper was slightly elevated above background level at 1.19 μg l-1.
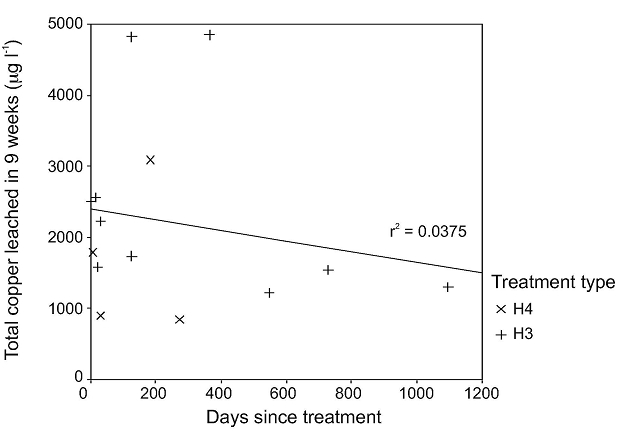
Figure 5. Copper leached from treated wood blocks of varying ages in nine weeks. Line of best fit is for H3 timber.
DISCUSSION
The use of CCA timber in caves has the potential to limit the number of cave organisms present if the component metals are leached in sufficiently high concentrations. Under certain conditions, CCA leachates are concentrated in the cave environment. Environmental conditions may also affect the rate of leaching.
Controlled laboratory experiments indicate that leaching rates of both arsenic and copper from treated wood are generally lower in karst waters than in distilled water. This suggests estimates of leaching rates obtained in non-karst waters should be considered as pessimistic estimates of leaching rates in karst waters.
Recharge waters in Ruakuri and Glowworm caves contain negligible arsenic, indicating no natural contribution of arsenic to the caves. The higher level of arsenic (sample 24 - 1.6 μg l-1) obtained for recharge water in Ruakuri cave probably leaches from a large volume of farm rubbish in a doline on the surface, 60m above the cave. By dye tracing, water was observed to take approximately two weeks to travel from the doline to the sampled drip point in the cave. Arsenic in the Mangawhitikau Cave stream leaches from a weir constructed in the cave using H3 and H4 timber in November 1999. The arsenic concentration of the stream immediately downstream of the weir was 0.57 μg l-1 in November 2001, whilst the copper concentration was 0.35 μg l-1. The volume of the weir is approximately 0.83m3, with a surface area of 26.6m2. The concentrations observed are equivalent to an annual flux of approximately 45g arsenic and 27g copper a year, assuming mean stream discharge of 250 l s-1. These figures compare with a median arsenic concentration in the Waikato river at the Whakamaru tailrace for the period 1996-2000 of 29 g l-1, derived from geothermal sources (Environment Waikato, 2002). It is estimated that two years after the weir was intalled, the leaching rates were 1.2% a-1 of the 0.37kg arsenic, and 1.0% a-1 of the 0.27kg copper in the weir. These low rates of leaching are due to the age of the timber (about 2 years), and reflect the rapid decline in leaching rates experienced in the first few months of environmental exposure, observed in the laboratory analysis of treated wood of various ages.
The source of arsenic and copper in the caves examined here is cave structures such as bridges, walkways and handrails. Where water flows rapidly over this timber, the arsenic and copper concentrations in the water are not significant. However, where CCA timber is placed in standing water, arsenic may accumulate to concentrations toxic to some freshwater epigean crustaceans. This has implications for the use of treated wood in caves in situations where standing water is common. In addition, the use of freshly treated wood in caves is not recommended because the highest rate of leaching occurs immediately following treatment.
Original moisture content of the wood appears to have little effect on the leaching rate of arsenic and copper from treated wood. However, both arsenic and copper leach less readily in karst water. Arsenic leaches more from H3 wood and least from H5 wood, whilst copper leaches most from H5 wood and least from H3 wood. This situation is remarkable because the same solution was used to treat timber to all the hazard class standards - only the concentration of the solution was altered in the different treatments.
An important aspect of leaching from CCA timbers is the volume of the metals remaining in the timber following a long period of leaching. Leaching rates are highest in the first few days of timber treatment, rapidly falling to very low levels. In controlled experiments, an average of 16% (± 7%, n=20) of the arsenic and 2% of the copper in H3 wood leached in nine weeks (maximum rates were 34% and 5% respectively). In the same period an average of 2% of the arsenic and 3% of the copper in H5 wood leached from the timber (maximum rates 3% and 7%).
However, analysis of timber treated up to 3 years previously indicates that in the outside environment, arsenic leaching rates from H3 wood may increase in the long term, whilst copper leaching rates decline. This may be the result of inadequate treatment in previous years, or may reflect an underlying tendency for arsenic to become more mobile through time. Insufficient data was obtained to investigate H4 of H5 wood for the same patterns.
CONCLUSIONS
In laboratory experiments, arsenic and copper leach at similar rates from wood with different initial moisture contents. The metals leach at different rates from wood treated to different hazard class standards. Although the same solution is used to treat the different hazard class standards (at different concentrations), copper leaches more from H5 wood than from from H3 wood, whilst arsenic leaches more from H3 wood than from H5 wood. In nine weeks, an average of 16% arsenic leached from H3 treated wood, compared to just 2% of the copper. This higher level of arsenic leaching in H3 treated timber is not related to wood type, treatment plant, test protocols or environmental conditions, since all timbers were obtained from the same stock, treated identically with the same preservative solution and the results were obtained from a statistically large population. Further investigation of this phenomenon is warranted.
The metals examined here leach at significantly higher rates in distilled water than in karst water, particularly over long time periods. This indicates that experimental results obtained in terrestrial non-karst settings may be assumed to represent pessimistically the behaviour of CCA metals in cave/karst environments. Over periods of several months to years, the rate of leaching declines rapidly, but leaching of arsenic and copper still occurs, even after three years of exposure to the weather.
Leaching of CCA metals in the cave environment occurs at levels that are detectable in surrounding environmental waters. However, the potential impact on cave/karst organisms is limited when large volumes of water are available to dilute any CCA leachate. The most significant impacts occur where treated timber is placed in standing water. Arsenic and copper concentrations in standing water containing treated timber in the Ruakuri Cave reached levels poisonous to freshwater crustaceans and algae respectively.
As the volume of CCA timber used in individual cave structures increases, As and Cu leachates may rise to toxic concentrations. If timber structures are in contact with standing water for long periods of time, concentrations toxic to cave organisms may develop rapidly. Management of cave structures should include provisions for water drainage from around the timber, to prevent wood remaining in contact with standing water. A common practice in show caves is the stockpiling of timber from retired structures in out-of-sight areas. Unless this timber is protected from water, toxic concentrations of arsenic and copper may arise locally. It is recommended that CCA timber be removed from caves when structures are retired from service.
REFERENCES
Adler Ivanbrook, L., Breslin, V.T., 1999. Accumulation of copper, chromium, and arsenic in blue mussels (Mytilus edulis) from laboratory and field exposures to wood treated with chromated copper arsenate type C. Environmental Toxicology and Chemistry 18, 213-221
Bennett, J.P., Chiriboga, E., Coleman, J., Waller, D.M., 2000. Heavy metals in wild rice from northern Wisconsin. Science of the Total Environment 246, 261-269
Bodek, I., Lyman, W.J., Reehl, W.F., Rosenblatt, D.H., 1988. Environmental Inorganic Chemistry, Pergamon Press, New York
Brown, C.J., Eaton, R.A., 2001. Toxicity of chromated copper arsenate (CCA)-treated wood to non-target marine fouling communities in Langstone Harbour, Portsmouth, UK. Marine-Pollution-Bulletin 42, 310-318
BSI, 1994. Wood preservatives - methods of measuring losses of active ingredients and other preservative ingredients from treated timber - part 2: Laboratory method for obtaining samples for analysis to measure losses by leaching into water or synthetic seawater. British Standards Institute, London
Canivet, V., Chambon, P., Gibert, J., 2001. Toxicity and bioaccumulation of arsenic and chromium in epigean and hypogean freshwater macroinvertebrates. Archives of Environmental Contamination & Toxicology 40, 345-354
Dickson, G.W., Briese, L.A., Giesy, J.P., Jr., 1979. Tissue metal concentrations in two crayfish species cohabiting a Tennessee, USA, cave stream. Oecologia 44, 8-12
Environment-Waikato, 2002. Arsenic in the Waikato River
http://www.ew.govt.nz/ourenvironment/water/healthyrivers/waikato/wormgraphs/arsenic.htm. Environment Waikato, 19-3-2002
Erry, B.V., Macnair, M.R., Meharg, A.A., Shore, R.F., 2000. Arsenic contamination in wood mice (Apodemus sylvaticus) and bank voles (Clethrionomys glareolus) on abandoned mine sites in southwest Britain. Environmental Pollution 110, 179-187
Franklin, N.M., Stauber, J.L., Markich, S.J., Lim, R.P., 2000. pH-dependent toxicity of copper and uranium to a tropical freshwater alga (Chlorella sp.). Aquatic Toxicology 48, 275-289
Garnacho, E., Peck, L.S., Tyler, P.A., 2000. Variations between winter and summer in the toxicity of copper to a population of the mysid Praunus flexuosus. Marine Biology (Berlin) 137, 631-636
Hedley, M.D., 1997. An assessment of risks associated with use of CCA-treated timber in sensitive environments and options for its substitution with alternative timber materials. Conservation Advisory Science Note 154, Department of Conservation, Wellington
Herkovits, J., Helguero, L.A., 1998. Copper toxicity and copper-zinc interactions in amphibian embryos. Science of the Total Environment 221, 1-10
Hess, R.E., Blanchar, R.W., 1977. Dissolution of arsenic from waterlogged and aerated soil. Journal of the Soil Science Society of America 41, 861-865.
Hingston, J.A., Collins, C.D., Murphy, R.J., Lester, J.N., 2001. Leaching of chromated copper arsenic wood preservatives: a review. Environmental Pollution 111, 53-66
Huang, P.M., Liaw, W.K., 1978. Distribution and fractionation of arsenic in selected fresh water lake sediments. International Revue de Geosciences et Hydrobiologie 63, 533-543
Knauer, K., Behra, R., Hemond, H., 1999. Toxicity of inorganic and methylated arsenic to algal communities from lakes along an arsenic contamination gradient. Aquatic Toxicology 46, 221-230
Lussier, S.M., Gentile, J.H., Walker, J., 1985. Acute and chronic effects of heavy metals and cyanide on Mysidopsis bahia. Aquatic Toxicology 7, 25-35
Mason, R.P., Laporte, J.-M., Andres, S., 2000. Factors controlling the bioaccumulation of mercury, methylmercury, arsenic, selenium, and cadmium by freshwater invertebrates and fish. Archives of Environmental Contamination & Toxicology 38, 283-297
Mastin, B.J., Rodgers, J.H.J., 2000. Toxicity and bioavailability of copper herbicides (Clearigate, Cutrine-Plus, and copper sulfate) to freshwater animals. Archives of Environmental Contamination & Toxicology 39, 445-451
Matschullat, J., 2000. Arsenic in the geosphere: A review. Science of the Total Environment 249, 297-312
Meador, J.P., 1991. The interaction of pH, dissolved organic arbon, and total copper in the detmination of ionic copper and toxicity. Aquatic Toxicology 19, 13-32
Ondov, J.M., Quinn, T.L., Han, M., 1996. Size-distribution, growth and deposition modeling of trace element bearing aerosol in the Chesapeake Bay airshed. Chesapeake Bay and Watershed Programs CBWP-MANTA-AD-96-1, Maryland Department of Natural Resources
Perschbacher, P.W., Wurts, W.A., 1999. Effects of calcium and magnesium hardness on acute copper toxicity to juvenile channel catfish, Ictalurus punctatus. Aquaculture 172, 275-280
Takamatsu, T., Aoki, H., Yoshida, T., 1982. Determination of arsenate, arsenite, monomethylarsonate, and dimethylarsinate in soil polluted with arsenic. Soil Science 133, 239-246
Weis, J.S., Weis, P., 1992. Transfer of contaminants from CCA-treated lumber to aquatic biota. Journal of Experimental Marine Biology and Ecology 161, 189-199
Weis, J.S., Weis, P., 1996a. The effects of using wood treated with chromated copper arsenate in shallow-water environments: A review. Estuaries 19, 306-310
Weis, J.S., Weis, P., 1996b. Reduction in toxicity of chromated copper arsenate (CCA)-treated wood as assessed by community study. Marine Environmental Research 41, 15-25
Weis, P., Nosker, T.J., Greenberg, A., Weis, J.S., 1992. Toxicity of construction materials in the marine environment: A comparison of chromated-copper-arsenate-treated wood and recycled plastic. Archives of Environmental Contamination & Toxicology 22, 99-106
Weis, P., Weis, J.S., 1999. Accumulation of metals in consumers associated with chromated copper arsenate-treated wood panels. Marine Environmental Research 48, 73-81